Disposal of Medical Wastes From Infectious Hospitalized Patients: A Mandate for Re-Examining Current Practices
Deborah Saber, PhD, RN, CCRN-K
University of Maine School of Nursing
Assistant Professor of Nursing
Abstract
This article explores the need for continued research on the solid waste disposal processes used in caring for infectious hospitalized patients. Current disposal processes were implemented in the 1980s when the focus was on preventing contagion from human blood and blood contaminated products. Medical solid waste challenges have evolved over the last three decades, and now include a need to address the spread of multi-drug resistant organisms (MDROs), increasing complexity of conditions and occurrence of co-morbidities that require multiple procedures for each patient, and a growing use of disposable patient care products that increase the waste stream exponentially. Developing strategies for minimizing the spread of infectious diseases will facilitate modernization of solid waste disposal processes to address emerging issues while supporting environmental health and sustainability.
“Lack of preparedness in medical waste management has other serious consequences beyond the potential spread of disease; shortfalls in disposal processes negatively impact the environment.”
When an infectious outbreak occurs, healthcare professionals are tasked with executing infection control processes that include waste management. These waste management procedures require a pre-determined, structured strategy. The 2014 Ebola outbreak in Texas underscored a lack of preparedness evidenced in infection control procedures and management and disposal of medical waste (Steenhuysen, 2014). Lack of preparedness in medical waste management has other serious consequences beyond the potential spread of disease; shortfalls in disposal processes negatively impact the environment. The protocols implemented to protect against microbial transfer via fomites from isolation units and isolated patients (operational orientation) may produce negative environmental impacts as compared to integrated indicators (i.e., dated waste disposal processes) (Little et al., 2016). Deleterious effects to the environment and community could result from an insufficient waste stream process where microbes can potentially transfer to many surfaces from the point of generation to the final treatment facility (Lubick, 2011).
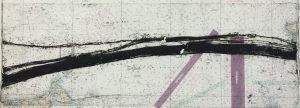
To improve medical preparedness, in November, 2014, President Obama requested 6.18 billion dollars to test for, treat, and prevent the spread of Ebola in hospitals. This budget included funds for staff training to address the logistics of medical waste removal (Daly, 2014). Support for developing procedures to effectively isolate individuals with dangerous infections – which included a waste management component – was provided by the Centers for Disease Control and Prevention (CDC; 2014, 2015) and U.S. Department of Health and Human Services (HHS; Koonin et al., 2015).
At the federal level, the Occupational Safety and Health Administration (OSHA) implements regulations to ensure the safety of workers on the job; individual states are authorized to define and regulate waste disposal processes for healthcare institutions operating within their borders. The Department of Transportation oversees the packaging and transportation of medical waste in Maine (Maine Department of Environmental Protection [Maine DEP], 2016). Solid waste is defined simply as solid materials that do not include biomedical or hazardous waste. According to Maine’s Biomedical Waste Management Rules (Maine DEP, 2016), biomedical waste “may contain human pathogens of sufficient virulence and in sufficient concentrations that exposure to it by a susceptible host could result in disease,” yet the rules provide no guidance for quantifying microbial virulence and no standard for evaluating the risk of contagion from medical solid waste (p. 2). Safe and efficient processing of biomedical waste mandates examination of current disposal processes to prevent the spread of specific microbes of concern and to address the issue of sustainability. This article highlights the need for focused examination of medical solid waste disposal protocols based on a convergence of factors including pathogen evolution (evidenced by increases in the number of microbes of concern); existing waste disposal process that are not specific for evolving infectious agents; increasing production of medical waste; growing complexity of patient profiles; and paucity of research focused on medical waste disposal processes, in developed nations, particularly from a sustainability perspective.
Increase and Changes in Microbes
The emergence of human immunodeficiency virus (HIV) in the 1980s focused attention on protocols for handling potentially infectious solid waste. The Medical Waste Tracking Act (MWTA) of 1988 imposed strict regulations on U.S. healthcare organizations in response to medical waste washing up on the east coast, an event that heightened public fears about the spread of HIV. Prior to implementation of MWTA there were just two classifications of hospital waste: solid waste contained in garbage bags and designated for municipal solid waste landfills, composting, or recycling, and biomedical waste containing disease-causing chemicals or infectious materials (Epstein, 2015).
The MWTA delineated new medical waste categories as follows:
- Cultures and stocks of infectious agents
- Human blood and blood products
- Human pathological wastes, including those from surgery and autopsy
- Contaminated animal carcasses from medical research
- Wastes from isolated patients with highly communicable diseases
- Used sharps (needles, scalpels, etc.) and certain unused sharps
(Fields, 1989)
- Dialysis and surgical waste
MWTA guided state waste disposal regulations and mandated disposal of patient care generated waste through the use of specific, standardized receptacles at a time when blood borne infections (e.g., HIV) were of extreme concern: red bags for biomedical waste; clear/white/black bags for general solid waste that is not classified as biomedical waste; and hard secured containers for sharp objects such as scalpels and needles. Like many states, Maine updated state guidelines by revising the basic definition of biomedical waste to include refuse associated with bodily fluids (e.g., pleural fluid, peritoneal fluid and fluids drained through canisters and tubing, dressings, disposable sheets, and blood). All of these liquids and patient care items have been found to carry infectious microbes (Chapter 900; Maine DEP, 2016). However, Maine’s updated disposal protocols do not delineate waste handling procedures based on the known or suspected presence of specific pathogens of concern. Wastes generated through caring for infectious patients are currently processed in the same manner as wastes from a non-infectious patient.
Classifications of pathogen virulence are defined by the National Institute of Allergy and Infectious Disease (2015). Today’s most feared pathogens are broadly classified in Category A, B, or C, information that must be considered in all discussions of medical waste (National Institute of Allergy and Infectious Disease [NIAID], 2015). Category A pathogens (e.g., Ebola, plague, anthrax, and smallpox) are of the greatest concern, with infected patients requiring the most intense caregiver preparedness. These agents pose an extreme risk to national security and to public health as they are easily disseminated, have a high mortality rate, and knowledge of infection has a propensity to cause panic and social disruption. Category B pathogens are moderately easily to disseminate, and may result in modest morbidity and low mortality (e.g., Hepatitis A, Salmonella). Pathogens in Category C can be engineered for mass distribution because of their availability, ease of dissemination, and potential high morbidity and mortality (e.g., influenza, multidrug resistant organisms [MDROs]), (NIAID, 2015). The surge of antibiotic resistant organisms such as methicillin-resistant Staphylococcus aureus (MRSA), vancomycin-resistant enterococci (VRE), penicillin-resistant pneumococci (PRP), and antibiotic-resistant gram negative bacteria is of increasing concern as the health care community confronts the emergence of a new generation of resistant organisms (Karpiak & Pugliese, 1991; Rao, 1989) and a growing variety of infectious diseases. Patients with MDROs are treated in contact isolation, where healthcare providers must wear disposable gowns and gloves in patient rooms to limit transfer to others. Isolation protocols acknowledge that MDRO infections are easily transferred, yet current waste disposal practices do not differentiate in treatment protocols for wastes from infectious and non-infectious patients; all waste (i.e., biomedical and general solid waste) is treated the same regardless of the infectious/noninfectious diagnosis of the patient.
“Solid waste from patients with MDROs impacts environmental sustainability in several ways. Additional waste is generated in caring for isolated patients (e.g., disposable gowns and gloves) when compared to non-isolated patients, and items are disposed of as general medical waste, which is then disposed of in landfills or incinerator and then sent to landfills.”
Increasing Waste Production
Since the inception of the MWTA, the quantity of waste generated in healthcare settings has increased dramatically. In 1991, Rutala and Weber found that hospitalized patients generated about 15 pounds of combined solid and medical waste per day. Considering total hospital waste (e.g., operating room disposables, food, pharmaceuticals, laboratory, patient care), the American Hospital Association ([AHA], 2015) estimated that patients could potentially generate 25 pounds each, or 60% more solid/medical waste per day, due in large measure to the increasing use of disposables. In addition, preliminary results (Saber et al., Unpublished Raw Data) from a study in one Maine hospital found that treating one MRSA-infected patient could produce 10.2 pounds of solid waste per day, 71.5 pounds of solid waste per week, or approximately 1.7 tons of solid medical waste per year. With increasing incidence of infectious disease (including infection with antibiotic resistant pathogens), and exponential growth in hospital waste production, it is clear that hospitals have a vested interest in developing safe, efficient, and sustainable protocols for processing, transporting, and disposing of biomedical and solid waste that is produced while caring for patients with infectious diseases (i.e., MDROs).
“The fundamental basis of sustainability depends on a healthy environment.”
Solid waste from patients with MDROs impacts environmental sustainability in several ways. Additional waste is generated in caring for isolated patients (e.g., disposable gowns and gloves) when compared to non-isolated patients, and items are disposed of as general medical waste, which is then disposed of in landfills or incinerator and then sent to landfills. The large quantities of waste could overload decontamination systems, creating interim storage challenges (Little et al., 2015). In addition, the fundamental basis of sustainability depends on a healthy environment. Infected waste that is improperly disposed of may present human and environmental risk factors as microbes are insidiously spread through fomites (David & Daum, 2010). The microbes, then, will continue to spread and pose environmental threats (CDC, 2013; David & Daum, 2010).
Complexity of Patients
Today’s hospital patient profiles contrast starkly with those of patients in the 1980s, most notably in the increasing complexity of health conditions. For example, in the 1980s comorbidities were limited and isolating patients with infectious diseases was not a routine practice. Today, isolation is standard procedure for patients with infectious diseases and a variety of comorbid conditions including hypertension, diabetes mellitus, Crohn’s disease, chronic obstructive lung disease, cancer, and drug and alcohol addiction. Enhanced technologies mandate the use of disposable supplies in treating these patients, who frequently require a greater number of bedside procedures, significantly increasing solid waste output. A major component of today’s medical waste stream includes disposables generated through direct patient care such as gowns, masks, and gloves, all of which are considered non-hazardous and are discarded in bags with unregulated waste (Saber et al., Unpublished Raw Data).
The Global Spread of Infection
Increasing global mobility presents significant opportunity for the spread of infectious agents (Tatem et al., 2006). The rate of transmission of highly contagious infections (e.g., Ebola, Avian Flu) and MDROs (Moulton et al., 2013; Zhou et al., 2014) is increasing exponentially. In 1968, during the last influenza pandemic, there were 261 million air travelers worldwide; in 2012, that number skyrocketed to nearly 3 billion annually (International Civil Aviation Organization, 2012; Warren et al., 2010).
Paucity in the Literature
A review of literature focused on disposal of medical wastes generated in caring for infectious patients in the U.S. highlights a deep gap in knowledge. This lack of literature is evidenced by searches of relevant databases such as Scopus, PubMed, CINAHL, Science Direct, Web of Science, Environmental Sciences and Pollution Management and others that yielded limited results (see Figure 1). Search criteria targeted research studies concerning management of infectious medical solid waste in U. S. health care facilities, such as hospitals or nursing homes, written in English. Because regulations differ between countries, those studies conducted outside the U. S. were excluded. A (2003) study conducted by Neely et al. examined the surface of waste disposal containers in patient rooms finding that single use containers were significantly less contaminated than reusable containers that had been cleaned. In a longitudinal investigation, Jagger et al. (2010), observed that more than 10% of sharps injuries to nurses and surgical technicians occurred during or after disposal; Perry et al. (2012) also investigated injuries involving sharps disposal and found that promptly replacing full sharps containers was critical in ensuring disposal safety. In a more recent study, Vatovec et al. (2013) used qualitative methods to investigate a variety of end-of-life care settings to identify the environmental and occupational and public health impacts of waste disposal on clinical practice. Researchers found that recycling efforts were limited by infectious waste contamination, including from reusable needle boxes harboring viable bacteria, and subsequently concluded that safe and effective waste disposal is an area of concern. The lack of literature on the topic of infectious solid waste disposal in the U.S. may be attributed to existence of a structured, regulated disposal process. These studies also indicate that disposal processes may not effectively protect health care workers or the community from emerging health challenges and suggest that re-examination of medical waste disposal processes is required.
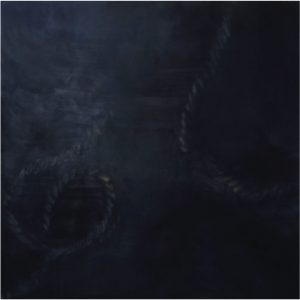
In the 1980s, the worldwide HIV contagion focused infection prevention strategies on bloodborne pathogens. Concerns today include HAI, MDROs, and highly contagious diseases that are transmitted through dermal contact and contact with bodily fluids other than blood. This increase in potential infection scenarios further highlights the need to update solid waste disposal guidelines for waste generated in caring for infectious patients.
There is a substantial body of hospital waste management research conducted in developing countries such as India (Kumar et al., 2014; Patil, & Pokhrel, 2005; Singh et al., 2014), Iran (Davoodi et al., 2014; Oroei et al., 2014; Taghipoura & Mosaferi, 2009), and Nigeria (Coker et al., 2009; Olukanni et al., 2014). But studies conducted in industrialized nations including Europe (Mühlich et al., 2003), the United Kingdom (Bates et al., 2008; Blenkharn, 2009), Italy (Giacchetta & Marchetti, 2013), and Portugal (Ferreira & Teixeira, 2010) are limited. Because U.S. waste disposal processes have long been defined, the lack of literature describing waste handling in the U.S. is not surprising.
Although regulated waste disposal systems exist in the United States today, deficiencies are apparent, based in part on changing patient pathologies. In 2010, an environmental survey conducted in 20 U.S. medical clinics found knowledge deficits among health care workers who were unsure of which solid wastes should be discarded in which containers (Savely et al., 2010). This study should inform the development of disposal protocols that will address two issues of equal concern: containing the spread of emerging infections and addressing sustainability concerns. A new model or algorithm delineating disposal protocols for solid wastes generated during infectious outbreaks (e.g., Zika virus, Chikungunya virus) is also needed.
Conclusions
Each year $120 billion is spent in the U.S. on frequently treated infectious diseases (Trust for America’s Health, 2014). These costs highlight the movement of infectious organisms once confined to healthcare facilities into our communities via fomites, including contaminated solid waste (David & Daum, 2010). Infection control is a significant problem as highlighted in a 2014 report evaluating American states’ capabilities to prevent, treat, and respond to infectious outbreaks. Five states scored eight (with 10 indicating the greatest capacity to prevent, treat, and respond), while 25 states scored five or less (Trust for America’s Health, 2014). Maine and seven other states scored 4/10. These findings indicate that most states are unprepared to deal with the spread of infectious diseases, including those currently monitored by state and federal agencies (CDC, 2015; Florida Department of Health [FDOT], n.d.; ProMED, 2015). In addition, burgeoning global travel has exacerbated the spread of disease including in rural and relatively unpopulated states such as Maine.
Medical waste disposal practices play an essential role in preventing the spread of disease, but current U.S. guidelines were developed more than two decades ago. Protocols implemented in 1988 cannot effectively address 21st century healthcare concerns such as the exploding use of disposable patient materials, the growing number and type of infections (e.g., MRSA, Vancomycin resistant enterococcus [VRE], Carbapenem-resistant Enterobacteriaceae [CRE], Klebsiella pneumoniae carbapenemase [KPC], Clostridium difficile [C. difficile]), and safe handling of wastes generated during treatment of highly infectious patients.
Failure to effectively manage medical wastes puts healthcare workers, sanitation workers, patients, and the public at risk for contracting infectious diseases (Floridahealth.gov, n.d.), facilitates propagation of drug resistant organisms, and drives escalating healthcare costs. Implementing a comprehensive, up-to-date infection control system that includes modern medical solid waste management strategies for healthcare facilities is critical to limiting the spread of drug resistant microorganisms, fostering sustainability by decreasing production of solid medical wastes, preventing pandemics, and reducing healthcare costs.
References
American Hospital Association. (2015). Sustainability roadmap for hospitals: A guide for achieving your sustainability goals. Retrieved 12 July, 2015 from: http://www.sustainabilityroadmap.org/topics/waste.shtml
Bates, M.P., Butler, S., Jones, K., Phillips, P.S., Tudor, T.L., & Woolridge, A.C. (2008). Utilizing a ‘systems’ approach to improve the management of waste from healthcare facilities: best practice case studies from England and Wales. Waste Management and Research, 26, 233-240. doi: 10.1177/0734242X07081482
Blenkharn, J.I. (2009). Sharps management and the disposal of clinical waste. British Journal of Nursing, 18, 860- 862. doi: 10.12968/bjon.2009.18.14.43353
Centers for Disease Control and Prevention. (2014). Hospital employee health. Retrieved 10 July, 2015 from: www.cdc.gov/niosh/topics/ohsn/pdfs/OHSNupdate.pdf
Centers for Disease Control and Prevention. (2015). Hospital preparedness: A tiered approach. Retrieved 10 July, 2015 from: www.cdc.gov/vhf/ebola/healthcare-us/preparing/current-treatment-centers.html
Coker, A., Sangodoyin, A., Sridhar, M., Booth, C., Olomolaiye, P., & Hammond, F. (2009). Medical waste management in Ibadan, Nigeria: Obstacles and prospects. Waste Management, 29, 804-811. doi: 10.1016/j.wasman.2008.06.040
Daly, R. (2014). Hospitals’ Ebola preparation costs can vary widely. Healthcare Financial Management, 68, 60-64.
David, M., & Daum, R. (2010). Community-associated methicillin-resistant Staphylococcus aureus: Epidemiology and clinical consequences of an emerging epidemic. Clinical Microbiology Reviews, 23(3), 616-687.
Davoodi, R., Eslami Hasan Abadi, S., Sabouri, G., Salehi, M., Ghooshkhanei, H.,
Rhamani, S., & Morshed, M. (2014). Medical waste management in the second
largest city of Iran (Mashhad) with three million inhabitants. Patient safety and Quality Improvement Journal, 2, 160-164. Retrieved 9 September, 2015 from: psj.mums.ac.ir/pdf_3401_4e01e70edf33fb9279a520bce4ce4a45.html
Epstein, E. (2015). Disposal and management of solid waste: Pathogens and diseases. Boca Raton, FL: CRC Press.
Ferreira, V. & Teixeira, M.R. (2010). Healthcare waste management practices and risk perceptions: Findings from hospitals in the Algarve region, Portugal. Waste Management, 30, 2657-2663. doi: 10.1016/j.wasman.2010.07.012
Fields, R. (1989). Containing waste disposal costs. Federation of American Health Systems Review, 22(5), 53-55.
Florida Department of Health. (n.d.). Disease reporting information for healthcare providers and laboratories. Retrieved 15 January, 2015 from: http://www.floridahealth.gov/diseases-and-conditions/disease-reporting-and-management/index.html
Giacchetta, G., & Marchetti, B. (2013). Medical waste management: A case study in a small size hospital of central Italy. Strategic Outsourcing: An International Journal, 6, 65-84. doi: 10.1108/17538291311316072
International Civil Aviation Organization. (2012). Annual passenger total approaches 3 billion according to ICAO 2012 air transport results. Retrieved 11 November, 2015 from: http://www.icao.int/newsroom/pages/annual-passenger-total-approaches-3-billion-according-to-icao-2012-air-transport-results.aspx
Jagger, J., Berguer, R., Phillips, E.K., Parker, G., & Gomaa, A.E. (2010). Increase in sharps injuries in surgical settings versus nonsurgical settings after passage of national needlestick legislation. Journal of the American College of Surgeons, 210, 496-502. doi: 10.1016/j.jamcollsurg.2009.12.018
Karpiak, J., & Pugliese, G. (1991). Medical waste: Declining options in the 90s. American Journal of Infection Control, 19, 8-15.
Koonin, L., Jamieson, D., Jernigan, J., Beneden, C., Kosmos, C., Harvey, M., & Damon, I. (2015). Systems for rapidly detecting and treating persons with Ebola virus disease – United States. Centers for Disease Control and Prevention: MMWR Morbidity and Mortality Weekly Report, 64, 1-4. Retrieved 17 June, 2015 from: www.cdc.gov/mmwr/pdf/wk/mm64e0303a2.pdf
Kumar, R., Gupta, A.K., Aggarwal, A.K., & Kumar, A. (2014). A descriptive study on evaluation of bio-medical waste management in a tertiary care public hospital of North India. Journal of Environmental Health Science and Engineering, 12, 69. Retrieved 9 September, 2015 from: http://www.ijehse.com/content/12/1/69
Little, J. C., Hester, E., & Carey, C.C. (2016). Assessing and enhancing environmental sustainability: A conceptual review. Environmental Science & Technology, 50, 6830-6845. doi: 10.1021/acs.est.6b00298
Lubick, N. (2011). Tools for tracking antibiotic resistance. Environmental Health Perspectives,119(5), 214-217.
Maine Department of Environmental Protection. (2016). Waste management: Biomedical waste. Retrieved 13 November, 2016 from: http://www.maine.gov/dep/waste/biomedical/index.html
Moulton, J., Tambyah, P., Ang, B., Ling, M.L.,& Fisher, D. (2013). The global spread of healthcare-associated multidrug resistant bacteria: A perspective form Asia. Healthcare Epidemiology, 56, 1310-131.
Mühlich, M., Scherrer, M., & Daschner, F.D. (2003). Comparison of infectious waste management in European hospitals. Journal of Hospital Infection,55, 260-268. doi: 10.1016/j.jhin.2003.08.017
National Institute of Allergy and Infectious Diseases. (2015). Biodefence and emerging infectious disease. Retrieved 10 October, 2015.
Neely, A., Maley, M., & Taylor, G. (2003). Investigation of single-use versus reusable infectious waste containers as potential sources of microbial contamination. American Journal of Infection Control, 31, 13-17.
Olukanni, D.O., Azuh, D.E., Toogun, T.O., & Okorie, U.E. (2014). Medical waste management practices among selected health-care facilities in Nigeria: A case study. Scientific Research and Essays, 9, 431 – 439. doi:10.5897/SRE2014.5863
Oroei, M, Momeni, M., Palenik, C.J., Danaei, M., & Askarian, M. (2014). A qualitative study of the causes of improper segregation of infectious waste at Nemazee Hospital, Shiraz, Iran. Journal of Infection and Public Health, 7, 192-198. doi: 10.1016/j.jiph.2014.01.005
Patil, G.V., & Pokhrel, K. (2005). Biomedical solid waste management in an Indian hospital: A case study. Waste Management, 25, 592-599. doi: 10.1016/j.wasman.2004.07.011
Perry, J., Jagger, J., Parker, G., Phillips, E.K., & Gomaa, A. (2012). Disposal of sharps medical waste in the United States: Impact of recommendations and regulations, 1987-2007. American Journal of Infection Control, 40(4), 354-358. doi: 10.1016/j.ajic.2011.04.328
ProMED. (2015). Retrieved 15 January, 2015 from: http://www.promedmail.org/
Rao, G. (1989). Risk factors for the spread of antibiotic-resistant bacteria. Drugs, 55, 323-330.
Rutala, W., & Weber, D. (1991.) Infectious waste: Mismatch between science and policy. The New England Journal of Medicine, 325, 578-582.
Saber, D., Waterman, T., Nichols, J., Nosel, E., Sears, S., & Dowd, K. [Solid waste and waste disposal processes for isolated patients with infectious disease: Preliminary results]. Unpublished raw data.
Savely, S., Hamilton, W., Degani, F., Weinberg, A., & Muraca, P. (2010). A survey of environmental safety issues at 20 medical clinics. Journal of Environmental Health, 73(6), 86-94.
Singh, A., Agarwal, A., Agarwal, V.K., Saxena, S.K., Agarwal, A.K., & Singh, H. (2014). Evaluation of bio-medical waste management practices in a tertiary care hospital of Rohilkhand Region in Uttar Pradesh, India. International Journal of Medical Science and Public Health, 3, 1187-1191. doi: 10.5455/ijmsph.2014.250620144
Steenhuysen, J. (2014, September 24). Insight: US hospitals unprepared to handle Ebola waste. Retrieved 10 December, 2014 from: http://www.reuters.com/article/2014/09/24/health-ebola-usa-hospitals-idUSL2N0RP00E20140924
Taghipoura, H., & Mosaferi, M. (2009). Characterization of medical waste from hospitals in Tabriz, Iran. Science of the Total Environment, 407, 1527-1535. doi: 10.1016/j.scitotenv.2008.11.032
Tatem, A.J., Hay, S.I., & Rogers, D.J. (2006). Global traffic and disease vector dispersal. Proceedings of the National Academy of Sciences, 103, 6242-6247.
Trust for America’s Health and the Robert Wood Johnson Foundation. (2014). Outbreaks: Protecting Americans from infectious diseases. Retrieved 11 September, 2015 from: http://healthyamericans.org/reports/outbreaks2014/
Vatovec, C., Senier, L., & Bell, M. (2013). An ecological perspective on medical care: Environmental, occupational, and public health impacts of medical supply and pharmaceutical chains. Ecohealth, 10, 257-267. doi: 10.1007/s10393-013-0855-1
Warren, A., Bell, M., & Budd, L. (2010). Airports, localities and disease: Representation of global travel during the H1N1 pandemic. Health & Place, 16, 727-735.
Zhou, Y., Wilder-Smith, A., & Hsu, L.-Y. (2014). The role of international travel in the spread of methicillin-resistant Staphylococcus aureus. Journal of Travel Medicine, 21, 272-281.
Figure 1: Literature Search