Jarvis Glacier Microstructure & Rheology
Understanding what controls glacial flow is important for making accurate sea level rise predictions. From experiments, we know that microscopic characteristics like crystallographic orientation, should affect the flow, but this has never been tested in a real glacier. This study will correlate glacial crystallographic orientation with strain rate.
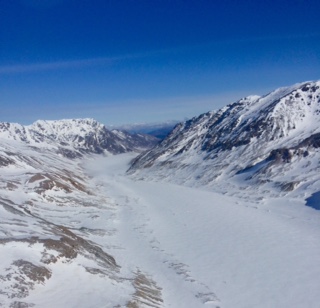
Project Overview
Glacier and ice sheet mass balance is one of the highest impact components of climate change, due in large part to its affect on sea level. Both Greenland and Antarctica, as well as alpine glaciers, discharge primarily though streaming flow, so the dynamics of that flow is central to the overall mass balance of the cryosphere. In glaciers and ice streams, the resistance to flow at the bed is important, but equally important is the internal viscous strength of the ice near the margins. In many cases, the lateral margins support >50% of the resisting stress. At present, there is moderate to high uncertainty of the factors controlling the viscous strength of streaming ice under natural conditions. We propose a plan to measure three-dimensional velocity, temperature, and microstructural parameters on Jarvis Glacier, in the eastern Alaska Range, in order to determine the relationship among microstructure, strain rate, and the constitutive laws necessary to numerically reproduce the observed kinematics.
To do this, we will first use surface velocity measurements, knowledge of the glacier geometry derived from ground penetrating radar, and numerical modeling to identify a site for drilling. We will then collect surface-to-bed cores across lateral and vertical strain rate gradients. Velocity and temperature measurements derived from the boreholes will complement the surface measurements and allow us to produce a more sophisticated three dimensional numerical model to test rheologic sensitivity within the study area. We will compare the microstructure (e.g., grain size distribution, crystallographic fabric) in the ice cores to the in-situ and modeled velocities and temperatures.
Project Goals & Methods
- Quantify the three-dimensional glacial geometry and surface kinematics. GPR and GPS.
- Model the internal kinematics to identify lateral and vertical strain rate gradients. Elmer/Ice.
- Collect surface-to-bed cores across selected strain rate gradients.
- Measure the temperature and strain rate distribution in the core holes. Freeze-in temperature and tilt sensors in two holes and multiple measurements of an open hole.
- Characterize the microstructural changes across the gradient. Cryo-stage SEM EBSD for crystallographic orientation. Thin sections and optical pictures for grain size.
- Evaluate the sensitivity of ice kinematics to observed microstructural and thermal variation. Elmer/Ice.
Collaboration with Kimberley Rain Miner who will be using portions of the Jarvis glacier ice core for her study:
Developing a risk assessment model for release of legacy pollutants in glacial outflows
Starting in 1950, organic pollutants in the form of pesticides and industrial byproducts were released into the atmosphere and distributed through precipitation onto alpine glaciers throughout the world. Recent abrupt climate change is increasing the melt rate of these glaciers, introducing the toxins to the watershed. While studies have demonstrated the existence of legacy pollutants within glaciated ecosystems, no one has used standard toxicological testing methods to assess the risk posed by pollutants when released in glacial outflows. Therefore, the goal of this study is to develop a framework to assess the conditions under which glacial release of persistent organic pollutants are a risk to the health of downstream communities.
Collaboration with University of Alaska: What role do glaciers play in terrestrial subarctic hydrology?
Personnel
Chris Gerbi – primary PI, University of Maine
Microstructure, rheology, numerical modeling
Seth Campbell – PI, University of Maine
GPR, velocity, field work safety and logistics
Bob Hawley – PI, Dartmouth College
Borehole geophysics
Peter Koons – PI, University of Maine
Numerical modeling
Karl Kreutz – PI, University of Maine
Ice core drilling, field work logistics
Stephanie Mills – graduate student, University of Maine
Microstructure, numerical modeling
Steve Bernsen – graduate student, University of Maine
GPR, velocity
Ian Lee – graduate student, Dartmouth College
Borehole geophysics
David Clemens-Sewall – graduate student, Dartmouth College
ice core handling, borehole logging
Mike Waskiewicz – ice core driller, U.S. Ice Drilling Program
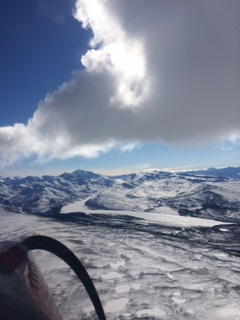
Project Timeline
Spring 2016 – GPR, stakes installed and initial GPS coordinates taken for velocity
Fall 2016 – second GPS measurements for velocity
Spring 2017 – GPR, velocity, drilling 3 ice cores, borehole measurements and installation of sensors, snow pits
Summer 2017 – analysis of microstructure, process GPR and velocity data
Fall 2017 – velocity, collect data loggers from frozen-in sensors, numerical modeling
2018 – numerical modeling
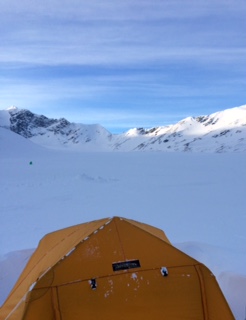