Five year post-installation review of a heat pump water heater
Thomas E. Stone1
1Husson University, 1 College Circle, Bangor, ME 04401
ABSTRACT
Heat pump water heaters extract energy from the surrounding air and transfer that energy to a water tank in order to heat the water. This is a very efficient process compared with more common hot water heating methods, and can be used to reduce a home’s overall energy consumption. Despite modest installation costs, which are currently mitigated by a state rebate program, and typical payback times on the order of three years, this technology is not widely adopted in Maine. Here I present a data set consisting of a central Maine family of four’s electrical usage for the five years prior to the installation of a heat pump water heater, and the five years after installation. I find that, on average, the home annually avoided 2,072 kilowatt-hours of electrical energy usage, 1,405 pounds of carbon dioxide emissions, and $311 in electricity costs after the heat pump water heater installation. Wider adoption of this technology would result in important environmental and economic impacts for individual families, and their collective action could also have large-scale implications.
1. Introduction
Of a home’s total energy consumption, approximately 14% is used for heating water (U.S. Energy Information Administration 2015a). Conservation of hot water, or any resource, will always provide the greatest ecological (and economic) benefit; honestly assessing and reconsidering our needs should always be the first action undertaken, second is then thoughtfully choosing to meet those needs in the most ecologically responsible way possible (Berry 2015). However, after all the end uses of hot water in a home have been truly reconsidered and minimized, the question then becomes how to most responsibly meet the remaining hot water demand. Some methods include electric resistive heating with a storage tank, gas with a storage tank or without (tankless), a heat pump water heater (HPWH), solar hot water heaters, and combinations of these methods. As the latest Intergovernmental Panel on Climate Change report, and others, makes clear, we must rapidly transition away from fossil fuels in order to mitigate the worst effects of climate change. This makes a hot water heater that minimizes fossil fuel consumption over its entire lifetime (including construction and disposal) the most desired option (Intergovernmental Report on Climate Change 2018, McGlade and Ekins 2015). Moore et al. (2017) modeled different methods of heating water in Australia and found that a solar hot water heater coupled with a HPWH powered from a solar photovoltaic (PV) array had the minimum greenhouse warming potential. However, solar hot water heaters, which use solar radiation to either directly or indirectly heat water and then store that hot water in a tank, are not widely used in Maine, likely due to higher upfront costs, structural concerns associated with the rooftop solar collectors, potential shading issues from trees, and general unfamiliarity with the technology (U.S. Energy Information Administration 2015b). Compared with the most widely utilized hot water heating methods in Maine (which do not include solar thermal and/or solar PV as a part of the system), Hong and Howarth (2016) found that a grid-powered HPWH produced lower greenhouse warming potential as a result of the heater’s use, though their calculations do not include emissions from the heater’s construction and disposal (U.S. Energy Information Administration 2015b). Using different assumptions, such as including the effects of refrigerant in HPWHs, Moore et al. (2017) calculated gas hot water heaters (both tank storage and on demand) to generate less greenhouse warming potential than a grid-powered HPWH over the entire lifecycle of each technology. These somewhat contradictory studies suggest that further life cycle analyses comparing HPWHs to gas technologies are required. However, both studies found that switching from a grid-tied electric hot water heater to a grid-tied HPWH (both with storage tanks) was advantageous with respect to minimizing greenhouse gas emissions as a result of operation and over the entire lifecycle; this is the comparison under consideration in this case study, though only emissions due to operation are calculated.
A HPWH consists of two resistive coils inside a storage tank with a heat pump integral to the unit (U.S. Department of Energy 2018, General Electric Appliances 2018, General Electric Appliances 2012). In the heat pump portion, a fan pulls air into the heat pump where thermal energy is extracted from the air and moved into the water via a refrigeration cycle. The resistive coils heat the water by passing an electric current through the coils as in a typical electric hot water heater. In heat pump mode the water is heated only with the heat pump whereas in electric mode the water is heated solely by the resistive coils. In hybrid mode, the heat pump will heat the water unless a low temperature setpoint is reached and the electric coils take over—this typically happens when the hot water demand is too great for the heat pump to maintain the desired water temperature and the coils are needed to more quickly return the water to the desired output temperature. Other modes of operation may exist depending on the particular model.
Due to the efficient heating of the water using the heat pump, switching to a HPWH is predicted to save 2,690 kWh1 of energy per year for a “typical” family of four (Energy Star 2018). In this case study, I present the actual energy savings by comparing residential electric data for both the five years prior to, and after, the installation of a HPWH in a central Maine home with four family members (two adults at the beginning of this study, two adults and two children by the end). I present this data set in order to provide additional information for Maine homeowners that are considering how best to heat water. Of course, the benefits and drawbacks of all water heating options should be considered and will vary by household—it is my hope that the results and references presented here, though limited by only considering one home, will prompt some homeowners to research HPWHs further.
2. Installation and Operation
In August 2013 I installed a 50 gallon General Electric Geospring hybrid hot water heater in an unheated basement directly beneath our home’s kitchen (General Electric 2018, General Electric 2012, Advanced Energy 2011).2 The retail price of $1,050 was reduced to $450 after taking advantage of then-current state and federal rebates—similar models currently cost ~$1,000 – $2,000, and qualifying HPWHs are eligible for a $750 state rebate (Efficiency Maine 2019a). The location of the old electric hot water heater met all of the recommended clearance and space requirements of the HPWH, so I simply removed the old heater and installed the new HPWH in its place, utilizing the existing electrical and water connections. I performed the installation myself (with help from more qualified family members) and so did not incur an additional cost of a plumber installing the machine (which is difficult to determine because plumbing costs can vary greatly, but might be on the order of $300).
Typically, I leave the HPWH in hybrid mode, though on days when hot water demand is exceptionally high I place the HPWH in electric mode in order to speed up recovery (though this is rare, perhaps 10 days a year). I am always conscious of the slower recovery time when in hybrid mode, which naturally leads us to spread out hot water demand throughout the day in order to avoid lukewarm water, but I have not attempted to further decrease our overall demand beyond that at the beginning of the 10 year period. If demand becomes too high for the heat pump to keep pace, the electric coils will turn on and the subsequent increase in water temperature can immediately be felt—a more comprehensive explanation of the various modes and temperature setpoints can be found in the HPWH technical service manual (General Electric 2012). The nominal hot water temperature setpoint was fixed at 125° F throughout this study.
Spreading out our hot water demand throughout the day (such as by setting the dishwasher to run overnight) has been the only long-term behavior adopted. More thoughtfully engaging with our home’s hot water demand might be considered a drawback to installing the HPWH, and in practice going to the basement to (very occasionally) switch modes or perhaps waiting an extra 15 minutes before taking a shower while the water temperature fully recovers from laundry and dishes can be mildly frustrating (a contributing factor is likely purchasing a tank size that is too small for our family). However, these are minor inconveniences compared to the environmental and economic benefits described in section 3. In fact, better understanding, interacting with, and questioning our household hot water demand as a result of installing a HPWH can be argued to also be a benefit. A more comprehensive listing of the benefits and drawbacks of a HPWH, as well as other water heating methods, can be found online through a number of sources, such as Efficiency Maine and the U.S. Department of Energy, or by contacting a trusted local retailer (Efficiency Maine 2019b, U.S. Department of Energy 2018, Shapiro and Puttagunta 2016).
The only maintenance likely to be undertaken by the homeowner is to clean the air filter periodically, when alerted by a warning alarm from the machine. This simply entails removing the filter, easily accessible on the top of the unit, and cleaning it with hot water. For more complicated repairs, a 10 year limited warranty appears to be the industry standard for HPWHs. In the last 5 years, the only time that the heat pump in the HPWH under consideration here was unable to function (hot water was still provided by the electric coils) was a condition in which the temperature sensor in the evaporator determined that the evaporator was not frost-free. The company’s customer service representative was unable to give us the name of a qualified repairman in the area, but was able to send a new fan assembly since that was one possible cause of the error code. I replaced the fan and associated wiring, clearing the fault. However, the fault continued to come in periodically that month, as well as in the same month a year later, always clearing within a day or so on its own. I conjecture that no repairs to the machine were actually necessary, but that the environmental conditions in our basement were too cold and wet during those months to allow for proper operation (ambient temperature must be 45° F – 120° F for the heat pump to function); the fault has not returned for 2 years.
3. Results and Discussion
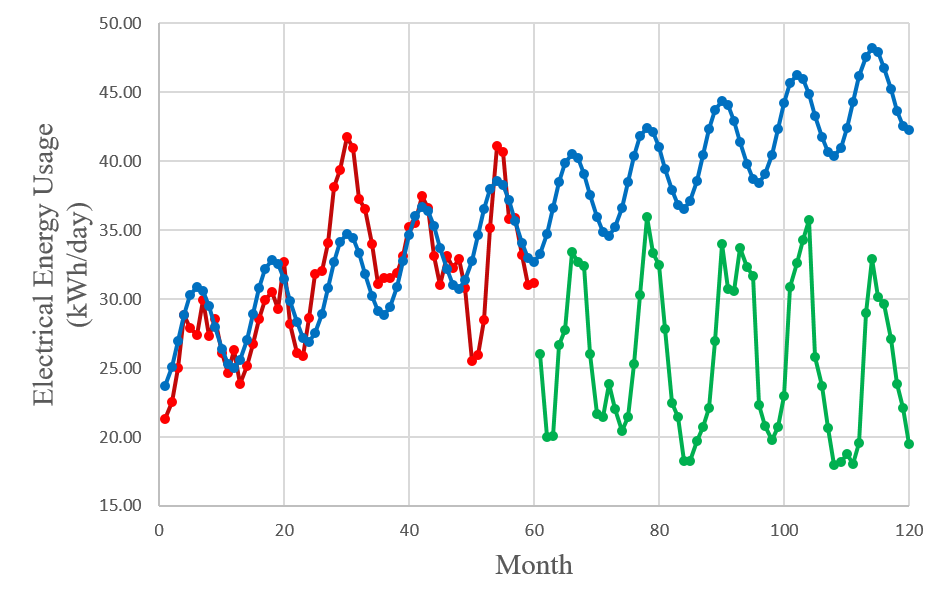
Figure 1 shows the average daily electrical energy use3 (kilowatt-hours per day, or kWh/day) for each month in the five years prior to installing the HPWH (red data) and for the five years after installation (green data).4 Each data point was calculated by taking the electrical energy (kWh) purchased each billing cycle and dividing by the number of days in the billing cycle—this information is readily available on the monthly electric bill, and should be accessible online in tabulated form as well. Average daily energy consumption was significantly lower after HPWH installation (t116 = 5.897, p < 10-7 for a two-sample t-test), a reduction from 31.3 ± 4.9 kWh/day to 25.7 ± 5.6 kWh/day (where the error bars indicate the sample’s standard deviation). Due to the observed 18% drop in electrical energy consumption correlating with the installation of a HPWH, I postulate that the HPWH was the primary reason for this decrease. This 18% reduction corresponds to a 5.6 ± 1.9 kWh/day decrease at the 95% confidence level.
In the five year pre-installation period, where an electric hot water heater of unknown age (though dating prior to at least 2004) provided hot water heating, our household used a total of 57,187 kWh of electrical energy. In the five year HPWH post-installation period, we used 46,828 kWh, a decrease of 10,359 kWh. Annually, our average electrical energy usage decreased from 11,437 kWh to 9,366 kWh, an average of 2,072 kWh savings each year (18%). I currently pay $0.17 per kWh of electricity purchased, but that amount has risen over the ten years of this experiment. If I assume an average electricity price of $0.15 per kWh, the 2,072 kWh savings is equivalent to avoiding $311 in electricity costs annually. At the total purchase price of $450, the HPWH paid for itself in approximately 17 months.
It should be stressed that the decrease in energy usage in the five year post-installation period cannot be entirely attributed to the adoption of a HPWH. While no large, intentional changes to energy consumption patterns were made (except for installing the HPWH), there were certainly unknown changes that occurred and contributed—in unknown ways—to the results presented here. For example, while the basement where the HPWH is installed remains at a relatively constant temperature, weather effects may have played a minor role in increasing or decreasing energy usage. A child was born in month 19 and then another in month 54, both of which would tend to drive energy consumption up (which makes the resultant 18% decrease an even more robust result). A small outdoor heater to keep the chicken’s water from freezing has been used the last two winters, a chest freezer was added to the barn at some point during the ten years, some old appliances died and were replaced with more efficient ones, and a myriad of other variables contributed to the home’s energy consumption—a household is clearly not a laboratory, where single variables can be controlled and their effect measured, which is both a weakness and a strength to this data set. Due to the 18% average annual reduction in energy usage (clearly visible in Figure 1) that corresponds to the HPWH installation, we can postulate that the HPWH was the primary cause as it was the only “large” electrical change made around that time. However, future work should include a large study of homes in Maine that use HPWHs in order to quantify their effects in a statistically meaningful way.
In addition to energy and cost savings, the 2,072 kWh of electrical energy saved annually also has environmental benefits. In Maine, 0.678 lbs of CO2 are released per kWh of electricity generated for the grid, which means the 2,072 kWh of energy savings prevents 1,405 lbs of CO2 from being produced annually (ISO New England 2016). These results are presented in Table 1, including a per-capita correction that accounts for four people living in the household. It is instructive to place the 1,405 lbs of CO2 not released into context. Considering that the United States is projected to emit 5,237 x 106 metric tons of CO2 in 2018, or 1.15 x 1013 lbs, it is easy to consider 1,405 lbs as being insignificant (Lindstrom 2018). Even if everyone in the U.S. switched to a HPWH, we might expect to annually avoid generating (351 lbs CO2 / person)(3.3 x 108 people) ≈ 1011 lbs CO2, or ~1% of the country’s total CO2 emissions (U.S. Census Bureau 2018). It should be acknowledged that, even in the best case scenario, HPWH technology plays only a small role in addressing CO2 emissions. However, we should also not confuse small with insignificant. It is impossible to identify all of the positive changes that may result from a simple HPHW installation, but some might include 1) considering a solar PV array for electricity to power the HPHW and other electrical loads with less fossil fuels; 2) looking into additional heat pump technology for home heating and cooling; 3) exploring increased insulation and sealing to keep the home at the desired temperature with less energy inputs; 4) recognizing that greater local, state, or national support (such as via various incentive programs) would enable others to undertake similar home improvements, and petitioning those in government that could institute such changes; or 5) becoming involved in government to ensure environmental stewardship is of the foremost consideration in policy creation. Any of these actions by this homeowner, or others influenced by this homeowner, might be considered small, but taken in their totality can amount to real, substantive change for the better.
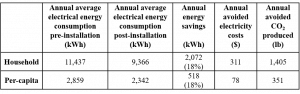
The data presented in Table 1 compares actual electrical energy consumption before and after the HPWH installation. It is also instructive to compare the predicted energy consumption after the installation with the actual energy consumption post-installation. The blue curve in Figure 1 is a mathematical model, based on the actual pre-installation energy consumption, that can be used to deduce trends in our energy consumption and predict future energy needs (see Appendix 1 for details). The cyclical nature of the curve reflects our greater electricity needs in the winter (more lighting, heating, inside cooking, etc.) and lesser needs in the summer (the home does not typically utilize air conditioning). More surprising was the linearly increasing nature of this model; some unknown factors were causing our average electrical usage to slowly increase over time, an observation that was not apparent until this data set was originally plotted. With so many variables in a household setting affecting electrical consumption, it is difficult to isolate a singular cause of this increase, but it is interesting to note that post-installation the expected cyclical pattern persists but the linearly increasing one does not.5 This suggests that the linearly increasing trend may have been due to the previous water heater failing over time.
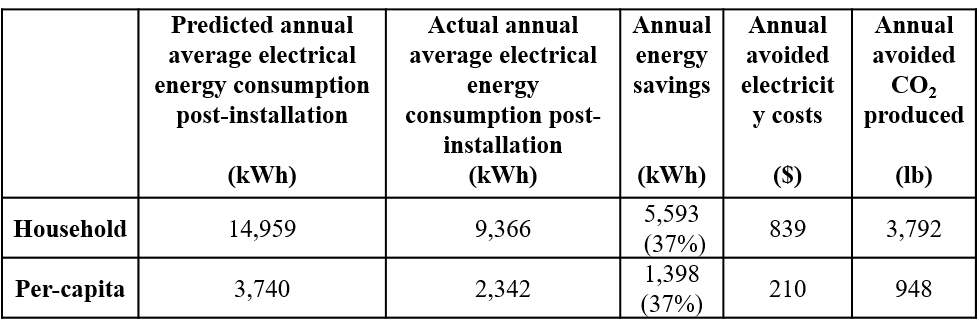
This simple model predicts a total electrical demand of 74,796 kWh over the 5 year post-installation period, or an average annual rate of 14,959 kWh. The predicted annual energy savings becomes substantially higher, 5,508 kWh, and the avoided economic and environmental costs follow suit (see Table 2). As mentioned in Appendix 1, we should use caution in relying too heavily on the predictions made by this model due to the complexity of the system—the 5,508 kWh predicted annual energy savings is more than double what a typical family is expected to see (Energy Star 2018). However, it is likely safe to regard the 2,072 kWh annual energy savings presented in Table 1 as being a conservative estimate of the actual energy savings provided by the installation of a HPWH in this home.
4. Conclusions
In this paper I have presented a 120 month data set consisting of a single home’s electric energy consumption before and after the installation of a heat pump hot water heater, in order to begin to quantify its performance. Using an actual home—with many unknown and uncontrolled for variables—does not allow us to conclusively say the energy reduction observed was due solely to converting from an older electric hot water heater to a hybrid hot water heater, though this conversion was the most likely cause since no other attempts (behavioral or structural) were made to reduce electric energy consumption. Keeping this in mind, I found that the home’s average annual electrical energy consumption decreased by 2,072 kWh after installation, which compares well with an industry estimate of 2,690 kWh. Furthermore, plotting the home’s energy consumption showed a previously undetected linear increase over time before the HPHW installation—reminding us of the value of trend analysis.
I conclude by reiterating that source reduction, in this case finding ways to lower overall hot water demand, should always be pursued first when trying to increase one’s environmental stewardship. Once that demand has been lowered as much as possible, it is my hope that the data set presented here will be helpful in assisting homeowners as they research various water heating options.
Footnotes
1. Units commonly encountered in the United States (kilowatt-hours, Fahrenheit, and pounds, for example) have been used throughout, instead of SI.
2. Note that this report is not an endorsement of a particular heat pump water heater and no such endorsement is implied. Though this report seeks to provide information to assist a homeowner in their research, responsibility for choosing a hot water heating option that best meets the home’s needs resides solely with the homeowner.
3. The HPWH does not provide any air heating for the home, which is heated primarily with a wood stove. In addition to the HPWH, the home’s electrical loading includes a washer, dryer, kitchen stove, dishwasher, refrigerator, lighting, and various plug loads.
4. The author will provide the data set upon request, and a copy of the data set accompanies this article.
5. It is not clear what is driving the magnitude of the variation around the mean (the standard deviation before installation is 4.9 whereas after installation it was 5.6). Due to the complexity of the system, there is no clear-cut reason why the post-installation variation is greater.
References
Advanced Energy. “GE Heat Pump Water Heater Report: January 7, 2011.”
https://www.peakprosperity.com/sites/default/files/content/document/files/geheatpumpwaterheaterfinaltestreportsealed.pdf (accessed January 23, 2019).
Berry, Wendell. “Less Energy, More Life.” Our Only World: Ten Essays. Berkeley: Counterpoint, 2015. 69-72.
Efficiency Maine. “Heat Pump Water Heater Rebate.” efficiencymaine.com (2019a).
https://www.efficiencymaine.com/at-home/heat-pump-water-heater-program/ (accessed January 23, 2019).
Efficiency Maine. “Heat Pump Water Heaters.” efficiencymaine.com (2019b).
https://www.efficiencymaine.com/at-home/water-heating-solutions/heat-pump-water-heaters/ (accessed January 23, 2019).
Energy Star. “Save Money and More with ENERGY STAR Certified Heat Pump Water Heaters.” energystar.gov (2018). https://www.energystar.gov/products/water_heaters/high_efficiency_electric_storage_water_heaters/benefits_savings (accessed December 30, 2018).
General Electric Appliances. “GeospringTM Heat Pump Water Heater.” geappliances.com (2018). https://www.geappliances.com/ge/heat-pump-hot-water-heater.htm (accessed December 30, 2018).
General Electric Appliances. “Technical Service Guide April 2012: GE Hybrid Water Heater (GEH50DEED).” https://www.manualslib.com/manual/767428/Ge-Geh50deed.html (accessed December 30, 2018).
Hong, Bongghi, and Robert Howarth. “Greenhouse gas emissions from domestic hot water: heat pumps compared to most commonly used systems.” Energy Science and Engineering 4, no. 2 (2016): 123-133. doi:10.1002/ese3.112.
Intergovernmental Report on Climate Change 2018. “Global Warming of 1.5 °C: An IPCC special report on the impacts of global warming of 1.5 °C above pre-industrial levels and related global greenhouse gas emissions pathways, in the context of strengthening the global response to the threat of climate change, sustainable development, and efforts to eradicate poverty.” ipcc.ch (2018). https://www.ipcc.ch/report/sr15/ (accessed January 23, 2019).
ISO New England. “2016 ISO New England Electric Generator Air Emissions Report.” https://www.iso-ne.com/static-assets/documents/2018/01/2016_emissions_report.pdf (accessed December 30, 2018).
Lindstrom, Perry. “U.S. energy-related CO2 emissions expected to rise slightly in 2018, remain flat in 2019.” eia.gov (2018). https://www.eia.gov/todayinenergy/detail.php?id=34872 (accessed January 23, 2019).
McGlade, Christopher, and Paul Ekins. “The Geographical Distribution of Fossil Fuels Unused When Limiting Global Warming to 2 °C.” Nature 517, no. 7533 (2015): 187-190. doi:10.1038/nature14016.
Moore, A.D., T. Urmee, M. Anda, and E. Walker. “Life cycle assessment of domestic heat pump hot water systems in Australia.” Renewable Energy and Environmental Sustainability 2, no. 38 (2017): 1-4. doi:10.1051/rees/2017043.
Shapiro, Carl and Srikanth Puttagunta. “Field Performance of Heat Pump Water Heaters in the Northeast.” U.S. Department of Energy (2016).
https://www1.eere.energy.gov/buildings/publications/pdfs/building_america/64904.pdf
(accessed December 30, 2018).
U.S. Census Bureau. “U.S. and World Population Clock.” census.gov (2018).
https://www.census.gov/popclock/ (accessed December 30, 2018).
U.S. Department of Energy. “Heat Pump Water Heaters.” energy.gov (2018).
https://www.energy.gov/energysaver/water-heating/heat-pump-water-heaters (assessed December 30, 2018).
U.S. Energy Information Administration. “2015 Residential Energy Consumption Survey (RECS).” eia.gov (2015a). https://www.eia.gov/consumption/residential/index.php (accessed January 23, 2019).
U.S. Energy Information Administration. “Water heating in homes in the Northeast and Midwest regions, 2015.” eia.gov (2015b). https://www.eia.gov/consumption/residential/data/2015/hc/php/hc8.7.php (accessed January 23, 2019).
Appendix
For the five years prior to HPWH installation, the average daily energy consumption (kWh/day) over each billing cycle (approximately monthly), when plotted as a function of time as in Figure 1, appears to linearly increase while being modulated by seasonal fluctuations. I thus postulate the following form for the daily energy consumption (E) as a function of time (t),
where the units of E are kWh/day and t is in months (t ≥ 1). The parameters {a,b,c,d} are to be determined; I immediately set the period of the sinusoidal modulation to T=12 since I anticipate those fluctuations to repeat yearly. The constants a = 26.51 and b = 0.16 are simply the y-intercept and slope of a linear regression through the data. The peak usage typically occurred within December, January, or February, so the constant d = 3 was next chosen so as to place the maximum of the sinusoidal piece in January of each year. The final constant, c = 3.44, was determined by minimizing the root mean square error between E(t) and the actual data. The final expression is
While this simple model captures the general patterns observed in the home’s electrical usage, care should be taken in relying too heavily on it for future predictions. First, it is not at all clear why the linearly increasing trend exists. This is a home, not a laboratory with variables that can be controlled singularly, and as such I can only speculate as to the reason. Since it is impossible to determine the trend’s cause, I cannot know how long into the future it might be anticipated to continue, if at all. Second, though the family’s only discernible behavior modification post-installation was to spread out the daily hot water demand, there may have been other changes undertaken that the family was not aware of, but that could have changed their electrical usage. Without fully understanding the underlying mechanisms driving the home’s electrical energy consumption, care must be used with the results from this model. Finally, developing a rigorous, statistically significant model is beyond the scope of this work. The model above is only intended to provide a first estimate of future electrical energy consumption.
The household energy use data used in this analysis is available here