Engaging Undergraduate Students in Local Stream Connectivity Issues by Measuring Environmental DNA from Anadromous Fish
By Kendra Harmon-Bolding1, Abbigail Hreben1, and Jason Kuhn2,‡
1 College of Science and Humanities, Husson University, Bangor ME
2 Assistant Professor of Chemistry, Husson University, Bangor ME
‡ Corresponding Author. Email: kuhnja@husson.edu. ORCid: https://orcid.org/0000-0002-0102-4448
Abstract
Many species of North Atlantic anadromous fish have experienced severe population declines in the past several decades. One major cause of these declines is believed to be reduced access to spawning streams caused by dams and other impoundments. In Maine, efforts are underway to increase migratory species’ access to historic watersheds by removing barriers and improving fish passage, with the goal of revitalizing Atlantic salmon and other anadromous fish populations. The use of environmental DNA can enable researchers to measure species presence in bodies of water, providing a fast and powerful tool to monitor population changes over time. Here, a single-semester undergraduate project is described in which students track select species using environmental DNA analysis. Before applying these methods to natural waterways, students first validated eDNA procedures using water samples from hatchery tanks which contained high levels of eDNA from a target species. The goal of this project is for students to participate in ecological monitoring in order to increase their awareness of local conservation issues.
Atlantic Salmon: A Migratory Species In Decline
The Atlantic salmon (Salmo salar) is a species of anadromous fish native to the North Atlantic. As anadromous fish, Atlantic salmon begin their lives in freshwater streams but spend most of their adult lives at sea. Adult Atlantic salmon can traverse hundreds of miles of river systems and ascend hundreds of feet in elevation in order to return to their natal streams to spawn during the autumn (Marschall 1998). Eggs are laid within constructed redds located in gravel streambeds, where the embryos incubate over winter before emerging in the spring as fry. These fry develop into parr over the next 2-4 years, then transition into seaward smolts in order to travel down the river system toward the ocean (Marschall 1998). At sea, adults mature for several years before returning to their natal freshwater streams to spawn, continuing the cycle of reproduction.
Salmon represent a key link between marine and freshwater systems, and are responsible for a massive transfer of marine nutrients into freshwater systems every year (Jonsson 2002). This nutrient influx has historically served as an important source of protein for animals and humans along riverine systems in North America where the range of Atlantic salmon rivers once extended from Quebec to Connecticut (Committee on Atlantic Salmon 2002). Like many of the 22 anadromous fish species in the North Atlantic, including the Alewife (Alosa pseudodharengus) and American shad (Alosa sapidissima), Atlantic salmon have suffered severe population declines over the past two centuries (Limburg 2009). In the United States, historic populations of Atlantic salmon include at least three distinct groups: the Long Island Sound population (extirpated in the early 19th century), the central New England population (extirpated in the mid 19th century), and the Gulf of Maine population, which is the only remaining population of wild Atlantic salmon in the United States (Committee on Atlantic Salmon 2002). Historically, this population likely produced 100,000 or more individuals per year; in recent years, however, the return of adult Atlantic salmon to spawn in Maine’s rivers has numbered in the hundreds or low thousands of individuals. In 2021, the Maine Department of Marine Resources reported a return of just 611 adults (Maine Department of Marine Resources 2022). Since 2000, the Gulf of Maine distinct population segment of Atlantic salmon have been listed as endangered under the Endangered Species Act (Committee on Atlantic Salmon 2002).
Global Challenges And Opportunities For Atlantic Salmon In Maine
The broad decline of anadromous fish species in the North Atlantic can be attributed to a complex interplay of global factors, including increased marine mortality, poor water quality, pressures from invasive/nonnative and aquaculture species, and especially loss of spawning habitat. All of these pressures are additionally compounded by stresses engendered by climate change (Limburg 2009).
One of the most significant threats to anadromous fish populations is caused by loss of spawning habitat, notably through the construction of dams, culverts, and other impoundments which disrupt river and stream connectivity. More than 1,000 known dams disrupt Maine’s waterways, with an unknown number of additional unregistered dams (American Society of Civil Engineers 2020). Dams adversely affect migratory fish species during both upstream and downstream passage; adverse effects include preventing or slowing fish passage, reducing water quality, altering local fish communities, and increasing direct and indirect mortality.
Dams pose significant barriers to anadromous fish migrating to spawning streams; without mechanisms to allow fish passage, dams can even sever species fully from upstream waters, such as the Medway Dam separating Atlantic salmon from the West Branch of the Penobscot River (Nieland 2020). Methods to allow fish passage include fish ladders, fish lifts, and trapping/ transporting individuals upstream. These methods have variable success for many migratory species, however, and even individuals that successfully navigate barriers can experience slower travel rates, with negative impacts on spawning success (Izzo 2016). Because each dam represents a unique barrier that invariably prevents passage for some individuals, the number of dams encountered, particularly on the main stem of a river as opposed to tributaries, has a high impact on the number of individuals that reach spawning streams. This is important because the majority of the highest quality salmon rearing habitat in terms of stream length and habitat quality lie toward the headwaters of streams, such as the East Branch of the Penobscot River (Fay 2006). As of 2024, in order to reach the East Branch of the Penobscot River, individuals must pass through no fewer than three dams [Milford at river kilometer (rkm) 63, West Enfield at rkm 101, and Weldon at rkm 149] while making use of a fish lift (rkm 63), vertical slot passage (rkm 101), and pool/weir passage (rkm 149) (Whittum 2022). Several studies have shown that Atlantic salmon migrating through dams on the Penobscot river with passage facilities demonstrate low cumulative passage efficiencies between 22 to 44% (Stevens 2018; Sigourney 2015).
In addition to posing formidable barriers to upstream passage, dams also increase direct and indirect mortality for adult salmon and smolts migrating downstream. Direct mortality events include injuries sustained due to passage through dam turbines or over fishways and bypasses. One study estimated a direct mortality to smolts migrating down the Penobscot of up to 9% per dam passed (Stevens 2018). Indirect mortality can result from passage delays, increased predation, and stress induced by moving through dams and reservoirs; stress can be compounded by alterations by dams to the properties and chemistry of local waters. By disrupting normal water flow, dams convert lotic systems into lentic systems, causing higher average temperatures and a concomitant lowering of dissolved oxygen in the reservoir system (Abbott 2022). Alterations in sediment and nutrient retention also impact water quality. The increase of lentic habitat can alter composition of fish communities and favor competing species such as largemouth bass (Micropterus salmoides), which prey on migrating smolts (Bae 2018).
Because of the significant role dams play in disrupting river connectivity and fish migration, improving fish passage or even removing dams entirely can yield improvements in stream ecologies and rates of returns. The Penobscot River Restoration Project (PRRP) was an effort to improve fish passage and remove dams on the largest salmon-bearing river in Maine; as part of this project, the Great Works (rkm 60) and Veazie (rkm 48) dams on the main stem of the Penobscot River were breached and removed in 2012 and 2013, respectively (Izzo 2016). Prior to removal of these dams, passage of Atlantic salmon was variably poor (as low as 43% at Veazie and 12% at Great Works Dam, depending on conditions) (Izzo 2016). Additional work was completed to improve fish passage for migratory species such as Atlantic salmon, Alewives, Blueback herring, Sea lamprey (Petromyzon marinus), and American shad (Alosa sapidissima); this was achieved in-part by installing an upgraded fish lift at the dam in Milford in 2014, now the first dam encountered by individuals travelling up the Penobscot River (Izzo 2016). Follow-up studies have confirmed that Atlantic salmon are now able to pass the Veazie and Great Works Dam remnants without delays, increasing their rate of movements from the ocean to the base of the Milford Dam (Izzo 2016). Another important benefit of removing these dams is the increased accessibility to cooler streams, such as the Great Works Stream; cooler streams can serve as thermal refuges during summer months, thereby helping to lower temperature-induced stress (Izzo 2016). The effectiveness of improved fish passage on Atlantic salmon movements has been mixed, however, as tagging studies have shown that individuals reaching the Milford fish lift can experience extensive passage delays. Only 35% of individuals reaching the fish lift in 2015 met the goal of being passed within 48 hours, and between 30 to 40% of individuals took more than a week to pass (Izzo 2016). Because passage delays at the Milford fish lift significantly interfere with the transit of Atlantic salmon along the lower stretches of the Penobscot River, addressing and improving these delays will be a necessary component if population numbers in the Penobscot River system are to recover.
Environmental DNA As An Emerging Tool For Species Monitoring
In order to understand the impacts of recovery efforts on anadromous fish, reliable methods must exist to track population numbers for species of interest. Electrofishing and weir/dam counts can be used to measure returning Atlantic salmon, while redd counts can shed light on spawning productivity. These methods can prove costly, time-intensive, and require specialized skills, and in the case of electrofishing, can stress or even injure animals. Environmental DNA (eDNA) analysis has proven to be a rapid, non-invasive method that allows researchers to determine species presence and abundance in different aqueous environments (Schadewell 2021). In the context of anadromous fish, eDNA is produced when individuals shed cells and secrete mucous, feces, and other organic particulates while passing through a body of water. These biological remnants contain cell-associated or soluble eDNA fragments, which can be collected in water samples. Cell, organelle, and eDNA fragments are trapped by passing water samples through filters, after which eDNA is extracted using DNA purification protocols (Schadewell 2021). Extracted eDNA can be amplified using the polymerase chain-reaction (PCR) along with species-specific DNA primers. The PCR method works by replicating eDNA molecules over a series of “cycles,” such that the number of eDNA molecules approximately doubles each cycle. Species-specific DNA primers restrict amplification of eDNA molecules to amplify only fragments from a specific target species, such as Atlantic salmon. Because this method can incorporate as many as 40 or more PCR replication cycles, a small number of starting eDNA fragments from the target species can generate an enormous number of DNA molecules following PCR replication, with detection limits as low as 10 eDNA molecules per liter sampled (Furlan 2016). In a version of PCR called quantitative PCR (qPCR), the amount of replicated DNA can be quantified each PCR cycle by incorporating a fluorescence tag which emits a fluorescence signal when it binds to double-stranded DNA molecules. Therefore, as the number of species-specific DNA molecules increases each PCR cycle, a higher number of fluorescent tags bind the DNA and generate a stronger fluorescence signal, which can be detected and quantified.
The use of eDNA as a proxy for species presence is well established and has been demonstrated for a wide variety of both aquatic and terrestrial species, including both prokaryotes and eukaryotes (Schadewell 2021). Many studies have used qPCR to measure eDNA from anadromous fish species, including Atlantic salmon and alewives (Atkinson 2018 ; Bradley 2022). While measuring eDNA has proven a robust method for detecting species presence, correlating eDNA measurements to numbers of individuals has proven more challenging. A number of factors complicate directly correlating between eDNA measurements and species populations, including eDNA shedding rates, sampling location/frequency/volume, hydrodynamic flow rates, and eDNA degradation rates, among others (Yates 2023). In the case of degradation, eDNA is susceptible to varying degradation rates within lentic versus lotic environments, freshwater versus marine environments, and in a temperature and pH dependent manner. Additional factors, such as the state of eDNA (i.e., whether it is intracellular, intraorganellar, surface-bound, or soluble) as well as environmental microbial load can also affect degradation rates (Jo 2021). Currently, much work is being done to better understand how eDNA measurements correlate with population numbers under different environmental conditions.
A One Semester Undergraduate Project to Engage Students in Local River Connectivity
Undergraduate classes that engage with environmental issues are correlated with increasing students’ pro-environmental attitudes and behaviors over the span of the class (Schmidt 2007 ; McMillan 2004). Further, this impact is amplified when classes focus on local environmental issues and incorporate student research experiences into the course’s design (Ascher 2018). In order to engage undergraduate students who are taking an upper-level Environmental Chemistry class in issues surrounding local water chemistry, stream connectivity, and migratory fish, a course-based research project was designed to be implemented over the span of one semester. This project uses eDNA as a readout for presence of anadromous fish species in various environments. Prior to sample collection, suitable sites were identified along the Penobscot River and its various tributary streams, including the Kenduskeag Stream near Bangor, Maine. The Penobscot River was chosen as a major focus along with several of its tributary streams because it is an easily-accessed, local river which contains the largest Atlantic salmon returns in the United States (with 1,310 adults counted at the Milford Dam fish lift in 2022) (Maine Department of Marine Resources 2022). Additionally, sampling sites along the Kenduskeag Stream were of particular interest because the Kenduskeag contains habitat suitable for Atlantic salmon spawning, and salmon redds have been sporadically detected in this stream as recently as 2019 (US Atlantic Salmon Assessment Committee 2020). Because individuals entering the Kenduskeag are not counted at the Milford Dam fish lift, samples collected from this body of water provide an ideal opportunity for students to explore the advantages and utility of eDNA detection in a novel context that warrants further study.
Numerous examples of eDNA extraction protocols exist in the literature; the protocol below was adapted from Atkinson et al. (2018). Briefly, one liter water samples are obtained at sampling sites and filtered using glass fiber filters with 1.5 micrometer pores. Distilled water samples are likewise filtered in order to serve as negative controls. Filters are stored at -20°C prior to DNA extraction. To extract eDNA trapped by the filters, filters are treated with cetyltrimethyl ammonium bromide (CTAB) extraction buffer containing 1 mg/mL Proteinase K and incubated for 2 hrs at 56°C. An equal volume of phenol/chloroform/isoamyl alcohol (25:25:1 v/v) is added, mixed, and centrifuged at 11,000 g for 20 minutes. The aqueous phase is then transferred into a new tube containing an equal volume of chloroform/isoamyl alcohol (24:1 v/v), mixed, and centrifuged again at 11,000 g for 20 minutes. The aqueous phase is again transferred into a new tube, and eDNA is precipitated by adding an equal volume of isopropanol to the aqueous phase, incubated at -20°C for 1 hour, then centrifuged at 20,000 g for 20 minutes. Pellets are washed with 70% ethanol and centrifuged for 5 minutes at 11,000 g. Ethanol is evaporated and pellets dried in a heating block for 5 minutes at 50°C before being resuspended in distilled water.
For qPCR experiments, forward and reverse primers specific to Atlantic salmon were ordered from Integrated DNA Technologies (Fwd: CGC CCT AAG TCT CTT GAT TCG A ; Rev: CGT TAT AAA TTT GGT CAT CTC CCA GA) (Atkinson 2018).
For PCR reactions, 30 microliter reaction volumes are used containing the following components: 15 microliters of PowerUp SYBR Green MasterMix, 3 microliters of forward and reverse primers, 3 microliters of isolated eDNA, and 9 microliters of distilled water. To measure amplified, double-stranded DNA, a QuantStudio 3 qPCR (Thermo Fisher) is used to quantify SYBR Green fluorescence (λ abs = 497 nm, λ em = 520 nm) after each cycle. qPCR settings include a 2-minute warm-up at 50°C, 10 min at 95°C, followed by 40 cycles between 95°C for 15 s and 60°C for 1 min (Atkinson 2018). To analyze eDNA results, students compare threshold values for fluorescence signals from environmental samples against a standard curve generated from serial dilutions of Atlantic salmon tissue-derived eDNA.
Prior to collecting river samples, eDNA extraction and qPCR methods were validated using water samples collected from Atlantic salmon tanks at Craig Brook National Fish Hatchery in East Orland, Maine. Additionally, water samples were obtained from Alamoosook Lake, which is adjacent to the hatchery, since water from hatchery tanks is ultimately cycled back into the lake. Because hatchery tanks contain stocks of Atlantic salmon, students have the opportunity to test qPCR methods on water samples which are expected to contain high concentrations of Atlantic salmon eDNA; in contrast, samples from Alamoosook Lake were expected to contain significantly lower Atlantic salmon eDNA compared to tank water. To quantify the amount of Atlantic salmon eDNA from these two sources, samples containing DNA extracted from known concentrations of Atlantic salmon tissue (1:100 serial dilutions between 1×10-4 and 1×10-12 g tissue per liter) were quantified along with a blank control using qPCR.
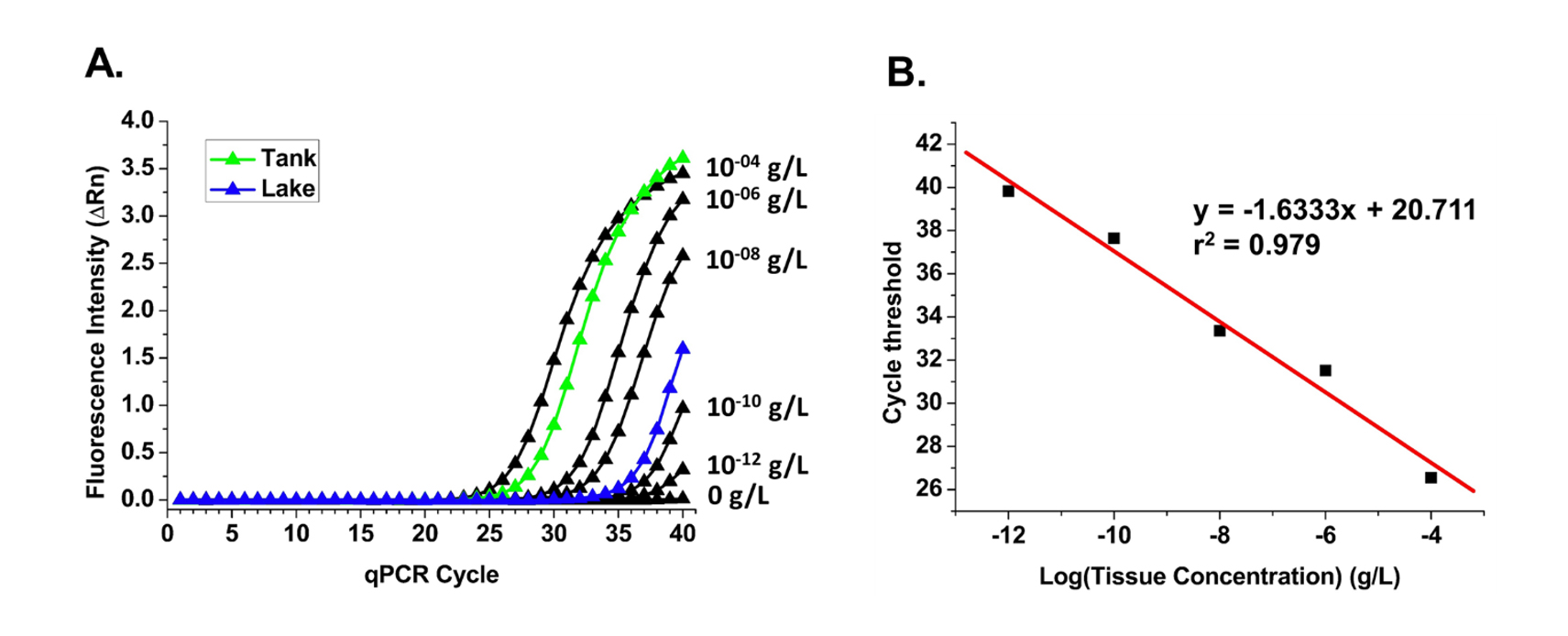
Students generated qPCR curves which showed that qPCR fluorescence signals correlate with concentrations of tissue standards (Figure 1A); eDNA extracts from higher tissue concentrations were detected at earlier qPCR cycles due to higher initial levels of DNA in these samples. qPCR curves were also generated using eDNA extracted from hatchery tank samples as well as from nearby Alamoosook Lake. The qPCR cycle at which each fluorescence signal rises above baseline (cycle threshold) was plotted against the log of the starting tissue concentration to generate a standard curve using known tissue standards (Figure 1B). Using linear regression and cycle threshold values, students calculated Atlantic salmon tissue concentrations for both hatchery tank water and Alamoosook Lake water samples (Table 1), showing that Atlantic salmon tissue concentrations (ie, eDNA from cells, waste, and other debris) were approximately 105 higher in hatchery tanks compared to the nearby lake.

These results show that the eDNA extraction methods and qPCR protocols are able to detect and quantify Atlantic salmon DNA from prepared tissue standards as well as samples obtained from hatchery tanks and a nearby lake. Following validation of methods using controlled samples, students next apply these methods to measure eDNA at different locations within the Penobscot River watershed, in which species presence and eDNA levels are expected to be more variable compared to hatchery samples.
Conclusion
The wide decline that many North Atlantic anadromous fish species have experienced over the last several decades illustrates the substantial impact humans have had on aquatic ecology. Several species that have suffered declines, such as Atlantic salmon, have historically played important ecological and cultural roles in the watersheds in which they return to spawn. One key driver of declining populations has been the loss of spawning habitat, which is especially driven by the disruption to river connectivity engendered by dams, culverts, and other impoundments. These disruptions can restrict or even sever anadromous fish from accessing the freshwater streams they need to spawn, with corresponding negative effects on reproduction. Encouragingly, efforts are underway in the United States and other countries to improve access to spawning habitat for Atlantic salmon and other anadromous species, such as through upgrading fish passage and removing dams, culverts, and other impoundments. The benefits of these efforts can be seen with returning anadromous species in watersheds such as the Penobscot River, where two dams, the Great Works Dam and the Veazie Dam, were removed in 2012 and 2013. Despite these improvements, many challenges still face Atlantic salmon and other anadromous fish species, and future recovery of populations will depend in part on continuing to improve access to spawning habitat. Engaging the public on issues of conservation is therefore an important component of future progress. To this end, a project suitable for a one-semester undergraduate class was designed. Using eDNA as a measure for species presence, students validated methods using hatchery water samples that contained high levels of Atlantic salmon eDNA; by comparing qPCR cycle threshold values for water samples against known tissue standards, students were able to determine tissue concentrations within hatchery tanks and Alamoosook Lake. After methods validation, students then apply these techniques to measure and map target species such as Atlantic salmon in poorly-characterized, local bodies of water. This project provides students with experience working with eDNA, which is an increasingly-valuable tool used in ecological monitoring and environmental sampling. Additionally, the project allows students to directly participate in local conservation issues and explore questions concerning stream connectivity and ecological health.
References
Abbott, K., Zaidel, P., Roy, A., Houle, K., Nislow, K. (2022) Investigating impacts of small dams and dam removal on dissolved oxygen in streams. PLoS ONE 17(11): e0277647.
Ascher, W. (2018) Safeguarding the enthusiasm for environmental studies: small is even more beautiful than before. Journal of Environmental Studies and Sciences, Springer; Association of Environmental Studies and Sciences, 8(1), 104-109.
Atkinson, S., Carlsson, J., Ball, B., Egan, D., Kelly-Quinn, M., Whelan, K., Carlsson, J. (2018). A quantitative PCR-based environmental DNA assay for detecting Atlantic salmon (Salmo salar L.) Aquatic Conservation: Marine Freshwater Ecosystems; 28: 1238-1243.
Bae, M., Murphy, C., Garcia-Berthou, E. (2018) Temperature and hydrologic alteration predict the spread of invasive Largemouth Bass (Micropterus salmoides). Science of The Total Environment, Volume 639, 2018, Pages 58-66, https://doi.org/10.1016/j.scitotenv.2018.05.001.
Bradley, D., Morey, K., Bourque, D., Fost, B., Loeza-Quintana, T., Hanner, R. (2022). Environmental DNA detection and abundance estimates comparable to conventional methods for three freshwater larval species at a power plant discharge. Environmental DNA, 4, 700–714. https://doi.org/10.1002/edn3.286
Committee on Atlantic Salmon in Maine, Board on Environmental Studies and Toxicology, Ocean Studies Board, National Research Council. (2002) Genetic Status of Atlantic Salmon in Maine: Interim Report. https://www.ncbi.nlm.nih.gov/books/NBK223890/pdf/Bookshelf_NBK223890.pdf
Fay, C., Bartron, M., Craig, S., Hecht, A., Pruden, J., Saunders, R., Sheehan, T., Trial, J. (2006) Status review for anadromous Atlantic Salmon (Salmo salar) in the United States. Report to the National Marine Fisheries Service, Silver Spring, Maryland and U.S. Fish and Wildlife Service, Falls Church, Virginia. https://repository.library.noaa.gov/view/noaa/4550
Furlan E., Gleeson D., Hardy C., Duncan R. (2016) A framework for estimating the sensitivity of eDNA surveys. Mol Ecol Resour. 16(3):641-654.
Izzo, L., Maynard, G., Zydlewski, J. (2016) Upstream movements of Atlantic salmon in the lower Penobscot river, Maine following two dam removals and fish passage modifications, Marine and Coastal Fisheries, 8:1, 448-461.
Jo T, Minamoto T. (2021) Complex interactions between environmental DNA (eDNA) state and water chemistries on eDNA persistence suggested by meta-analyses. Mol Ecol Resour. 2021; 21(5).
Jonsson, B., Jonsson, N. (2002) Migratory Atlantic salmon as vectors for the transfer of energy and nutrients between freshwater and marine environments. Freshwater Biology, Volume48, Issue 1, Pages 21-27.
https://www.maine.gov/dacf/lupc/projects/wolfden/review/ZP779A_Pickett_Mtn_ZoneChg_20230118.pdf
Limburg, K., Waldman, J. (2009) Dramatic declines in North Atlantic diadromous fishes. BioScience, Vol. 59 No. 11.
Maine Department of Marine Resources. (2022) Historical Trap Counts. https://www.maine.gov/dmr/sites/maine.gov.dmr/files/inline-files/Trap%20Count%20Archive%202022.pdf
Maine Section of the American Society of Civil Engineers. (2020) Infrastructure Report Card. https://infrastructurereportcard.org/wp-content/uploads/2021/07/FullReport-ME_2020_UPDATED.pdf
Marschall, E., Quinn, T., Roff, D., Hutchings, J., Metcalfe, N., Bake, T., Saunders, R., LeRoy Poff, N. (1998). A framework for understanding Atlantic salmon (Salmo salar) life history. Canadian Journal of Fisheries and Aquatic Sciences, 55(S1): 48-58. https://doi.org/10.1139/d98-007.
McMillan, E., Wright, T., Beazley, K. (2004) Impact of a University-Level Environmental Studies Class on Students’ Values, The Journal of Environmental Education, 35:3, 19-27, DOI: 10.3200/JOEE.35.3.19-27
Nieland, J., Sheehan, T. (2020) Quantifying the Effects of Dams on Atlantic Salmon in the Penobscot River Watershed, with a Focus on Weldon Dam. Northeast Fisheries Science Center Reference Document 19-16.
Schadewell, Y., Adams, C. (2021) Forensics meets ecology—environmental DNA offers new capabilities for marine ecosystem and fisheries research. Frontiers in Marine Science. 8:668822.
Schmidt, J. (2007) From Intentions to Actions: The Role of Environmental Awareness on College Students. UW-L Journal of Undergraduate Research X.
Sigourney, D., Zydlewski, J., Hughes, E., Cox, O. (2015) Transport, dam passage, and size selection of adult Atlantic salmon in the Penobscot river, Maine. North American Journal of Fisheries Management, 35:6, 1164-1176.
Stevens, J., Kocik, J., Sheehan, T. (2019). Modeling the impacts of dams and stocking practices on an endangered Atlantic salmon (Salmo salar) population in the Penobscot river, Maine, USA. Canadian Journal of Fisheries and Aquatic Sciences, 76: 1795-1807.
U.S. Atlantic Salmon Assessment Committee. (2020) Annual Report, Report No. 32 – 2019 Activities DOI : https://doi.org/10.25923/hmb7-hw09
Whittum, K., Zydlewski, J., Coghlan Jr, S., Hayes, D., Watson, J., Kiraly, I. (2022) Fish assemblages in the Penobscot river: A decade after dam removal. Marine and Coastal Fisheries: Dynamics, Management, and Ecosystem Science, 15:e10227.
Yates, M., Furlan, E., Thalinger, B., Yamanaka, H., Bernatchez, L. (2023) Beyond species detection—leveraging environmental DNA and environmental RNA to push beyond presence/absence applications. Environmental DNA; 5:829-835.