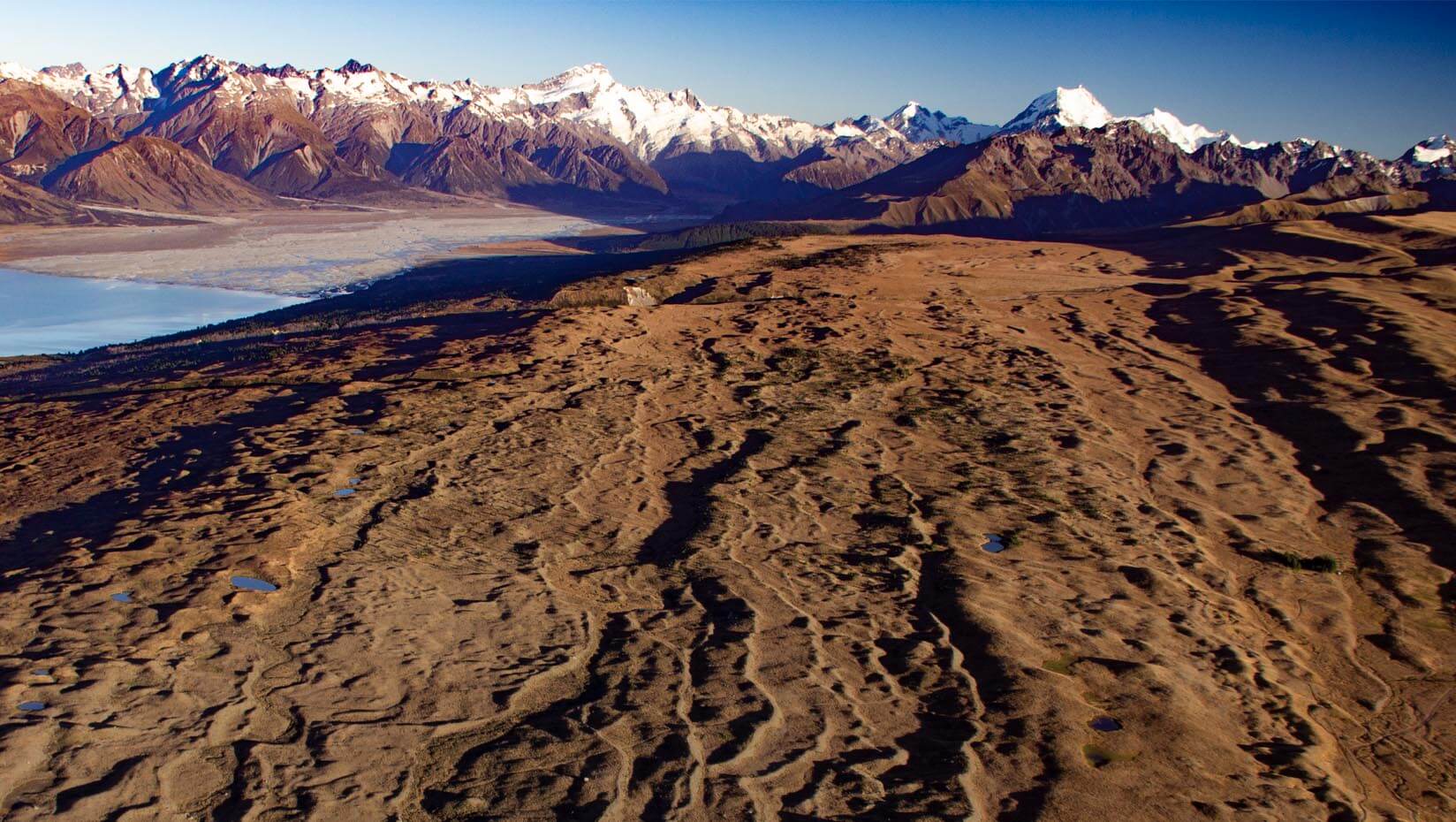
Zealandia Switch may be the missing link in understanding ice age climates
The origins of ice age climate changes may lie in the Southern Hemisphere, where interactions among the westerly wind system, the Southern Ocean and the tropical Pacific can trigger rapid, global changes in atmospheric temperature, according to an international research team led by the University of Maine.
The mechanism, dubbed the Zealandia Switch, relates to the general position of the Southern Hemisphere westerly wind belt — the strongest wind system on Earth — and the continental platforms of the southwest Pacific Ocean, and their control on ocean currents. Shifts in the latitude of the westerly winds affects the strength of the subtropical oceanic gyres and, in turn, influences the release of energy from the tropical ocean waters, the planet’s “heat engine.” Tropical heat spreads rapidly through the atmosphere and ocean to the polar regions of both hemispheres, acting as the planet’s thermostat.
The Southern Hemisphere climate dynamics may be the missing link in understanding longstanding questions about ice ages, based on the findings of the research team from UMaine, Columbia University’s Lamont-Doherty Earth Observatory, the University of Arizona, and GNS Science in New Zealand, published in Quaternary Science Reviews.
For more than a quarter-century, George Denton, UMaine Libra Professor of Geological Sciences, the journal article’s first author, has led research reconstructing the history of mountain glaciers in the Southern Hemisphere. In the late 1980s, he and Wallace Broecker, a geochemist at Columbia University, noted that a key question about ice ages remained unresolved — the link between ice age climate and the orbital cycles in the length and strength of the Earth’s season. Evidence showed that ice age climate changes were synchronous in both polar hemispheres, with rapid transitions from glacial to interglacial global climate conditions. They concluded that existing theories could not adequately account for changes in seasonality, ice sheet size and regional climate.
Mountain glaciers are highly sensitive to climate and well suited to climatic reconstruction, using distinctive moraine deposits that mark the former glacier limits. In the 1990s, Denton led research teams in the mapping and dating of moraine sequences in South America and, more recently, in New Zealand’s Southern Alps, with co-author David Barrell, geologist and geomorphologist with the New Zealand government’s geoscience research institute, GNS Science.
With advances in isotopic dating of moraines in the mid-2000s, Denton teamed up with Columbia University’s Joerg Schaefer, who directs the Cosmogenic Nuclide Laboratory at the Lamont-Doherty Earth Observatory. Together with CU-LDEO colleague and co-author Michael Kaplan, Schaefer, Denton, and UMaine assistant professor and co-author Aaron Putnam have guided a succession of UMaine graduate student field and laboratory projects (including Putnam’s Ph.D. work) that have developed a chronology of climate-induced glacier changes in the Southern Alps spanning many tens of thousands of years. The most recent participant in the UMaine-CU partnership is UMaine Ph.D. student and co-author Peter Strand.
Collectively, the UMaine, CU-LDEO and GNS Science partners have worked to create and compile mountain glacier chronologies from New Zealand and South America, producing a comprehensive chronology of glacier extent during and since the last ice age. The team then compared the moraine dating to paleoclimate data worldwide to gain insights into the climate dynamics of ice ages and millennial-scale abrupt climate events. The findings highlight a general global synchronicity of mountain-glacier advance and retreat during the last ice age.
Deep insights into the climate dynamics come from co-author Joellen Russell, climate scientist at the University of Arizona and Thomas R. Brown Distinguished Chair of Integrative Science. Following on her longstanding efforts at modeling the climatic modulation of the westerly winds, she evaluated simulations done as part of the Southern Ocean Model Intercomparison Project, part of the Southern Ocean Carbon and Climate Observations and Modeling initiative. The modeling showed the changes to the southern wind systems have profound consequences for the global heat budget, as monitored by glacier systems.
The “switch” takes its name from Zealandia, a largely submerged continental platform about a third of the size of Australia, with the islands of New Zealand being the largest emergent parts. Zealandia presents a physical impediment to ocean current flow. When the westerly wind belt is farther north, the southward flow of warm ocean water from the tropical Pacific is directed north of the New Zealand landmass (glacial mode). With the wind belt farther south, warm ocean water extends to the south of New Zealand (interglacial mode). Computer modelling shows that global climate effects arise from the latitude at which the westerlies are circulating. A southward shift of the southern westerlies invigorates water circulation in the South Pacific and Southern oceans, and warms the surface ocean waters across much of the globe.
The researchers hypothesize that subtle changes in the Earth’s orbit affect the behavior of the Southern Hemisphere westerly winds, and that behavior lies at the heart of global ice age cycles. This perspective is fundamentally different from the long-held view that orbital influences on the extent of Northern Hemisphere continental ice sheets regulate ice age climates. Adding weight to the Zealandia Switch hypothesis is that the Southern Hemisphere westerlies regulate the exchange of carbon dioxide and heat between the ocean and atmosphere, and, thus, exert a further influence on global climate.
“Together with interhemispheric paleoclimate records and with the results of coupled ocean-atmosphere climate modeling, these findings suggest a big, fast and global end to the last ice age in which a southern-sourced warming episode linked the hemispheres,” according to the researchers, whose work was funded by the Comer Family Foundation, the Quesada Family Foundation, the National Science Foundation and the New Zealand government.
The last glacial termination was a global warming episode that led to extreme seasonality (winter vs. summer conditions) in northern latitudes by stimulating a flush of meltwater and icebergs into the North Atlantic from adjoining ice sheets. Summer warming led to freshwater influx, resulting in widespread North Atlantic sea ice that caused very cold northern winters and amplified the annual southward shift of the Intertropical Convergence Zone and the monsoonal rain belts. Although this has created an impression of differing temperature responses between the polar hemispheres, the so-called “bipolar seesaw,” the researchers suggest this is due to contrasting interregional effects of global warming or cooling. A succession of short-lived, abrupt, episodes of cold northern winters during the last ice age are suggested to have been caused by temporary shifts of the Zealandia Switch mechanism.
The southward shift of the Southern Hemisphere westerlies at the termination of the last ice age was accompanied by gradual but sustained release of carbon dioxide from the Southern Ocean, which may have helped to lock the climate system into a warm interglacial mode.
The researchers suggest that the introduction of fossil CO2 into the atmosphere may be reawakening the same dynamics that ended the last ice age, potentially propelling the climate system into a new mode.
“The mapping and dating of mid-latitude Southern Hemisphere mountain-glacier moraines leads us to the view that the latitude and strength of the austral westerlies, and their effect on the tropical/subtropical ocean, particularly in the region spanning the Indo-Pacific Warm Pool and Tasman Sea through to the Southern Ocean, provides an explanation for driving orbital-scale global shifts between glacial and interglacial climatic modes, via the Zealandia Switch mechanism,” the research team wrote. “Such behavior of the ocean-atmosphere system may be operative in today’s warming world, introducing a distinctly nonlinear mechanism for accelerating global warming due to atmospheric CO2 rise.
Contact: Margaret Nagle, nagle@maine.edu