Current Research
Glacial record of the last termination event, Potanin Glacier Valley, Altai Mountains, Mongolia – Mariah Radue, Nathaniel Norris, Dr. Aaron Putnam
Field team members: Mariah Radue (University of Maine), Nathaniel Norris (University of Maine at Presque Isle), Peter Strand (University of Maine), Dr. Aaron Putnam (University of Maine), Destiny Washington (Gary Comer College Prep), Jessica Stevens (Gary Comer College Prep), Ninjin Tsolmon (Mongolian University of Science and Technology), Purev-Ochir Purevdorj (Mongolian University of Science and Technology), Scott Travis (Comer Family Foundation), Kevin Stark (Medill School of Journalism)
Funding support: National Science Foundation and Comer Family Foundation
Mongolian Altai, Mongolia
June 28-August 6, 2016
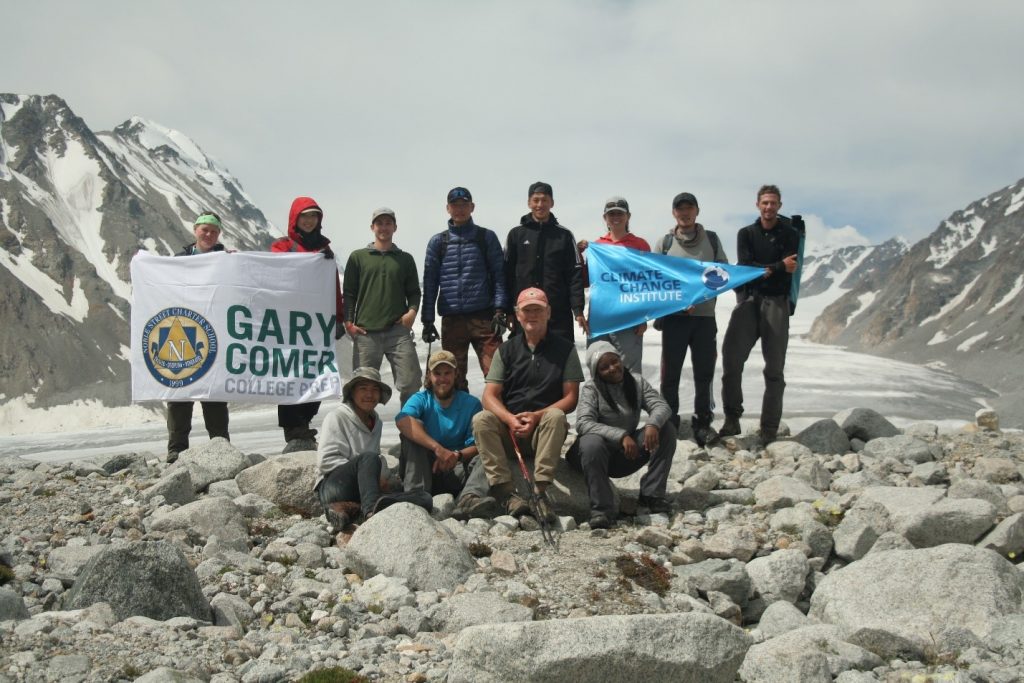
Research motivation: A chronology for the Potanin Glacier Valley in the Mongolian Altai will contribute to our understanding of climate in the interior of Asia during the Last Glacial Termination. The Potanin Glacier Valley is located approximately 45 km north of Khoton Lake, a region studied by Peter Strand and Dr. Aaron Putnam. We will create a 10Be surface-exposure chronology of glacial landforms along with surficial geomorphic maps to record the glacial history of the area. Our findings will help define the timing of deglaciation in the center of Earth’s largest continent and understand its relationship with increased CO2, radiative forcing, and tropical sea surface temperatures.
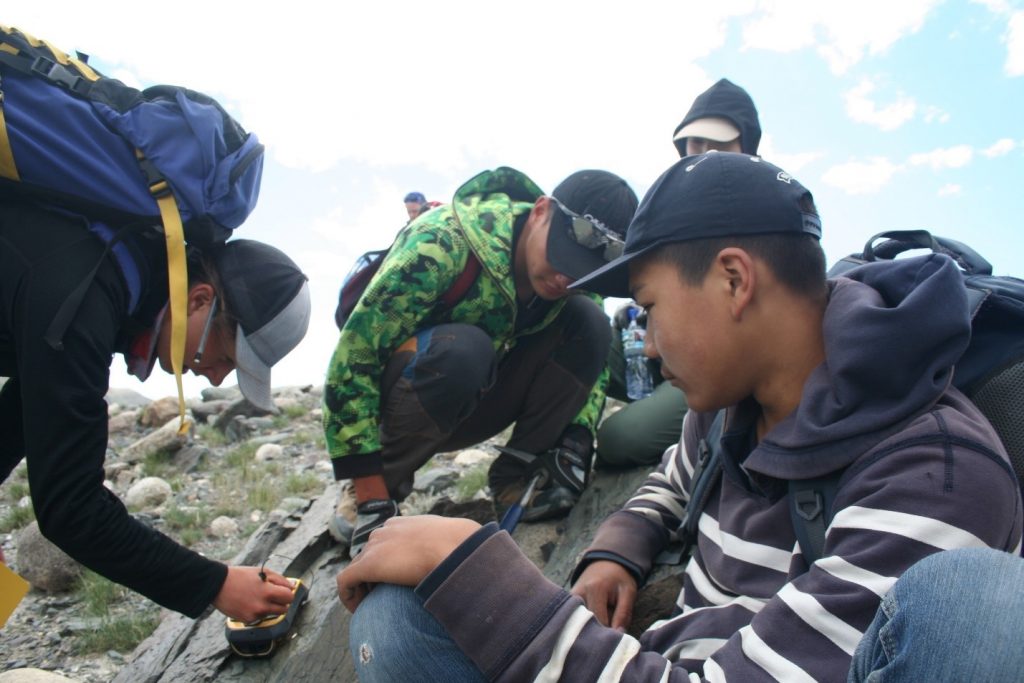
Field work: Our work in the Potanin Glacier Valley took place in three main locations – the Last Glacial Maximum moraine complex, Holy Mountain, a molded bedrock hill in the center of the valley, and Little Ice Age moraines. Mariah Radue (University of Maine) will use the former ice extents during the Late Pleistocene to track the rate of deglaciation. Nathaniel Norris (University of Maine at Presque Isle) will use ages obtained from Holy Mountain the create a history of ice thinning during deglaciation. Destiny Washington (Gary Comer College Prep) conducted geomorphic mapping of terminal moraines to quantify glacier lengths throughout time. In addition to surface exposure sampling, we employed a DJI Phantom 4 drone to take aerial photographs and create digital elevation models and orthomosaics. Students from the Mongolian University of Science and Technology (MUST) collected rock samples for petrological analysis to better understand the bedrock geology of the region.
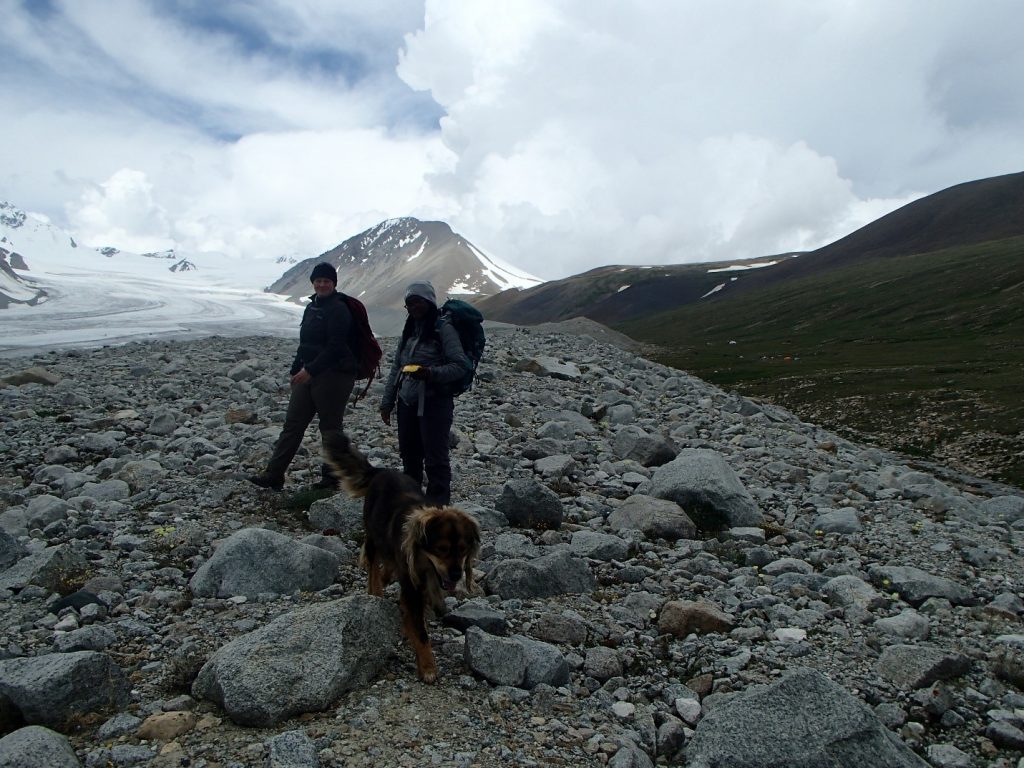
Thank you: We are grateful to Huvsgol Travel for their continuing help in logistics and permitting. Many thanks to Oyungerel Sambuu of MUST for helping with shipping and permitting as well as facilitating a connection between Mongolian and American students through field work. The Comer Family Foundation provided funding for Destiny Washington and her teacher Jessica Stevens of Gary Comer College Prep. This research was funded by the National Science Foundation.
A Beryllium-10 Chronology of the Last Glacial Termination in the Mongolian Altai – Peter Strand, Dr. Aaron Putnam
Field team members: Peter Strand (University of Maine), Dr. Aaron Putnam (University of Maine and Lamont-Doherty Earth Observatory), Dr. David Putnam and Caleb Ward (University of Maine Presque Isle), Sarah Kramer (Medill School of Journalism), Pagamsuren Amarsaikhan and Tsetsenbileg Bavuu (Mongolian University of Science and Technology), Tanzhuo Liu (Lamont-Doherty Earth Observatory), and Hayley Walcott (University of Saint Andrews)
Funding support: Comer Science and Education Foundation
Mongolian Altai, Mongolia
June 15 – July 25, 2015
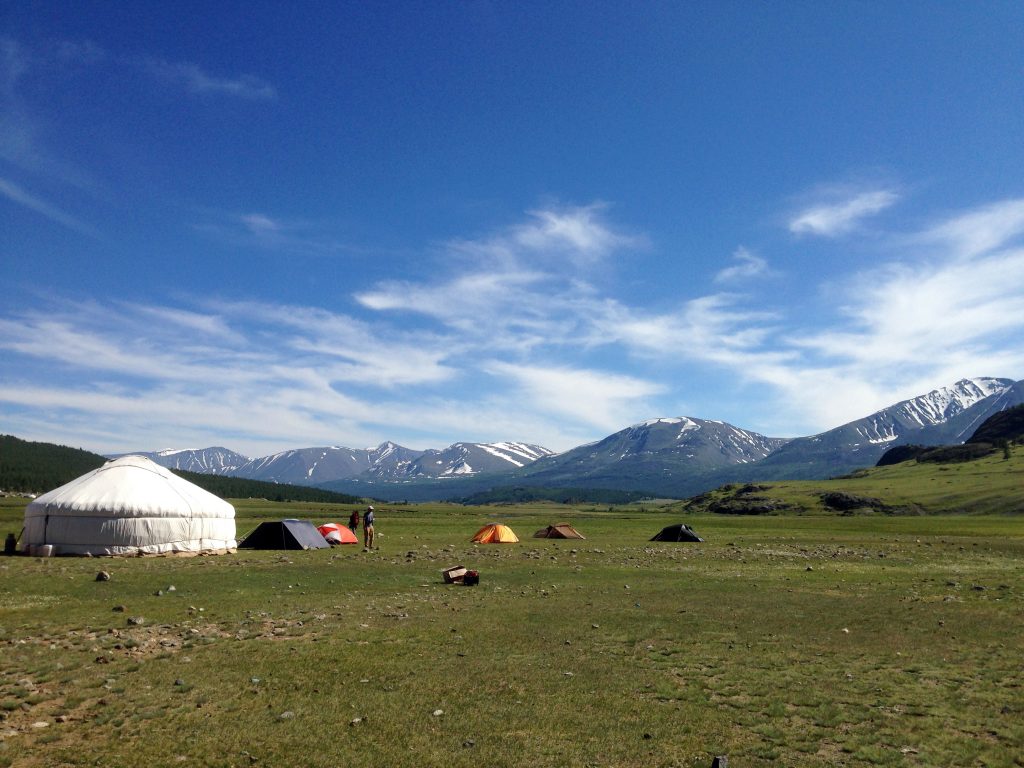
Research Motivation: The primary purpose of our expedition to Mongolia is to develop a 10Be surface-exposure chronology of glacial landforms corresponding to the end of the last ice age in the Mongolian Altai, at the heart of the Asian continent. Our fieldwork focused on the mapping and sample collection of moraines and glacial geomorphologic features around Khoton Nuur. Khoton Lake lies at the foot of the Altai Mountains near the Chinese border, at an elevation of about 2,000 meters (6,600 ft) above sea level. The collected samples will be processed at the University of Maine, and we will create a chronology documenting the reduction of glacier volume since the peak of the last ice age. The Altai Mountains lie at ~48°N latitude, and will therefore add a northern complement to our efforts undertaken in Southern Hemisphere mid-latitudes, e.g., New Zealand and Patagonia. The results will provide a test of recent hypotheses for glacial terminations.
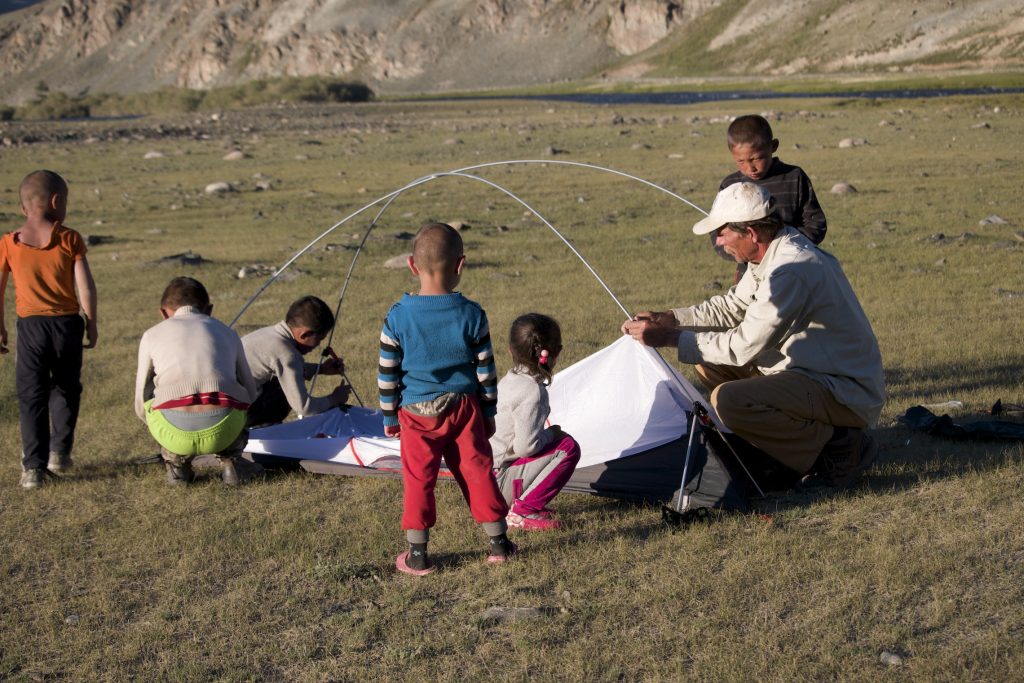
The last glacial termination represents the last great global warming and the last time CO2 rose by a substantial amount before the industrial period. And yet the role of CO2 in causing the last great global warming is not certain. A solution to this problem could help us to hone our understanding of the sensitivity of atmospheric temperature to CO2, as well as to inform our understanding of the processes that launch the earth out of an ice age. This research will also allow us to evaluate the relative roles of atmospheric CO2, insolation forcing, and tropical Pacific sea-surface temperatures in driving Northern Hemisphere warming to completion during the last deglaciation.
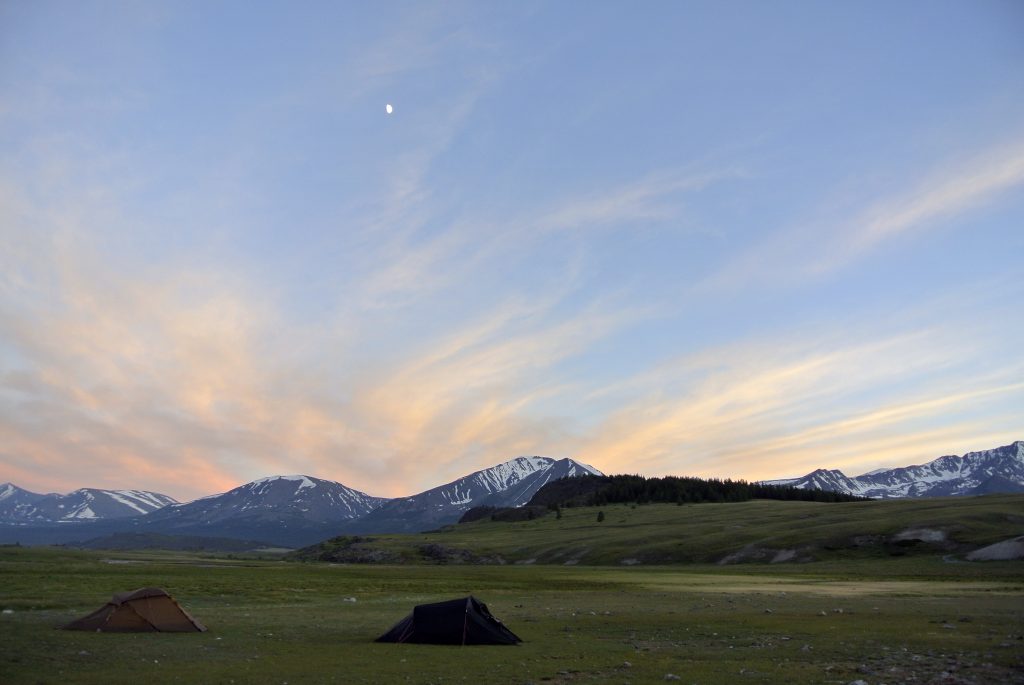
Field Work: We began our field season by camping up-valley of Hoton Nuur, near the Tungalag Gol (Crystal River). This campsite provided a centralized location for all field activities. We were able to rent a Kazakh ger from a local family, which served as our cooking and dining tent. Our wonderful camp cook, Chackie, did all the cooking along with her assistant Tomoroo. Chackie’s warm meals are a great treat after a long day of fieldwork. Aaron, Caleb, Sarah, Pagma, Tsetsee, Tomoroo and Peter were able to travel with local horses farther up the valley to the edge of the modern glacier. This trip was difficult, nevertheless, the team toughed it out and collected a suite of samples high up (~9,800 feet) in a glacial cirque valley. These samples will allow us to determine when the glacier’s snowline rose to pre-industrial values. We then moved down valley to the shores of Hoton Nuur. Our camp was situated between two large, glacially molded bedrock knobs, which provided the backdrop to many beautiful sunsets and sunrises.
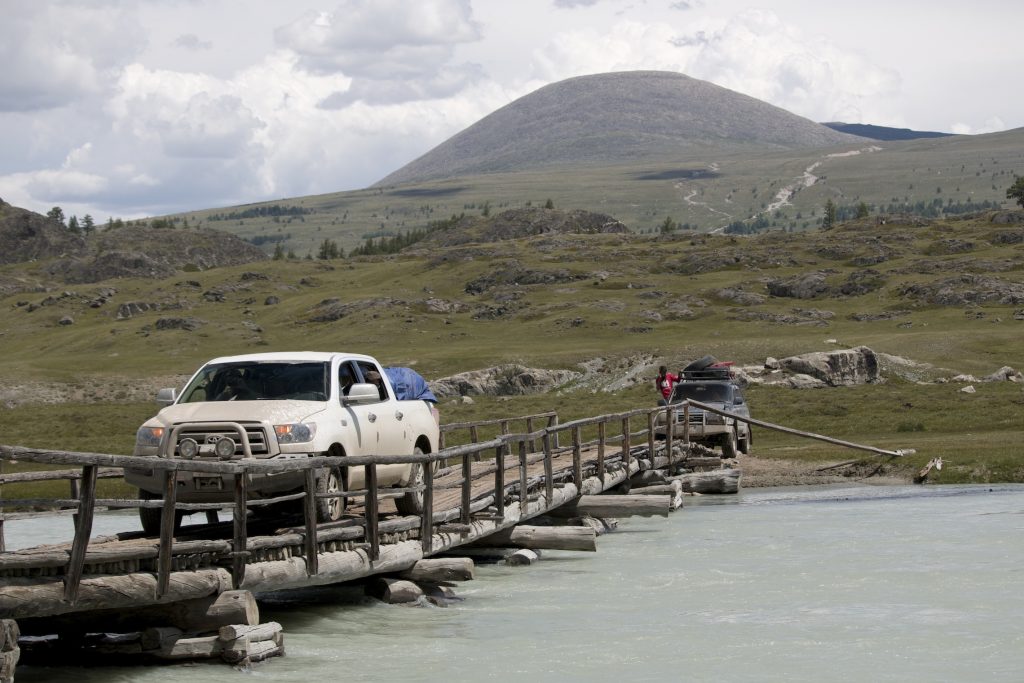
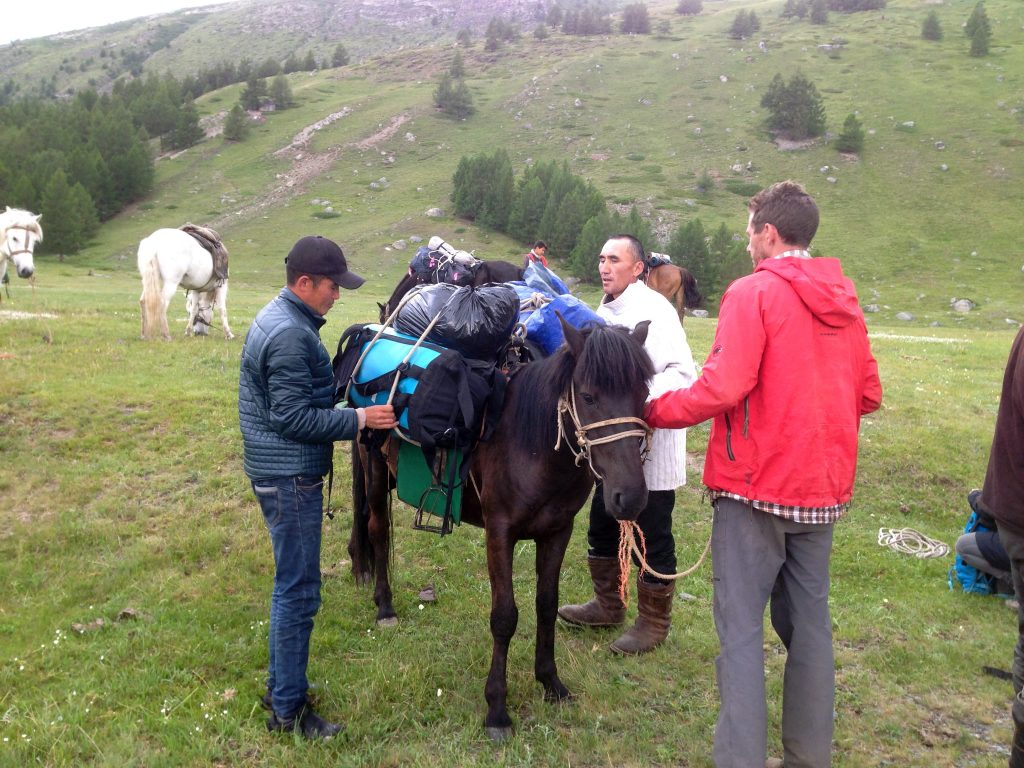
We mapped and sampled large erratic boulders that pepper the landscape and provide perfect targets for our sampling efforts. Once processed in the laboratory at the University of Maine, the deposition dates from these boulders will document when the Hoton Nuur glacier receded from the valley at the end of the last Ice Age. These data will further our understanding of the processes that launched the earth out of an ice age, and may even help to determine the sensitivity of atmospheric temperature to CO2 change. Caleb became a master of the ancient wedge-and-shim rock splitting technique used for rock sampling. Pagma and Tsetsee learned our sampling method in a heartbeat and became indispensable members. Even Tomoroo has learned the sampling ropes. The sampling effort continued at Hoton Nuur, where we are focusing on mapping and dating large moraine ridges that impound the lake.
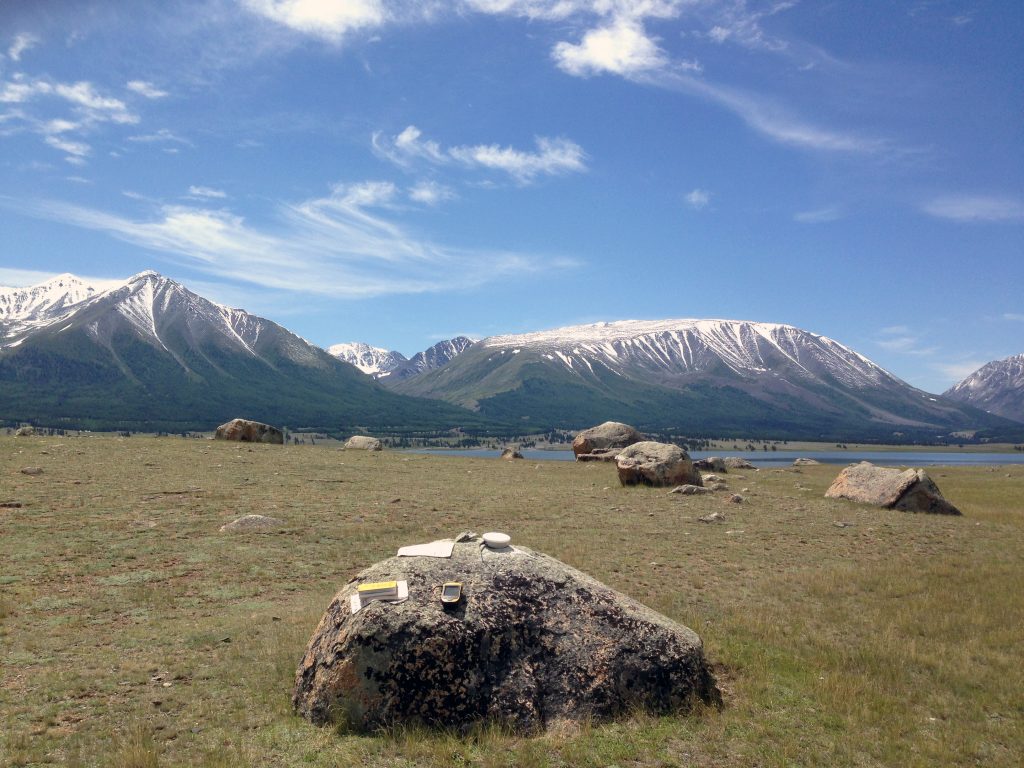
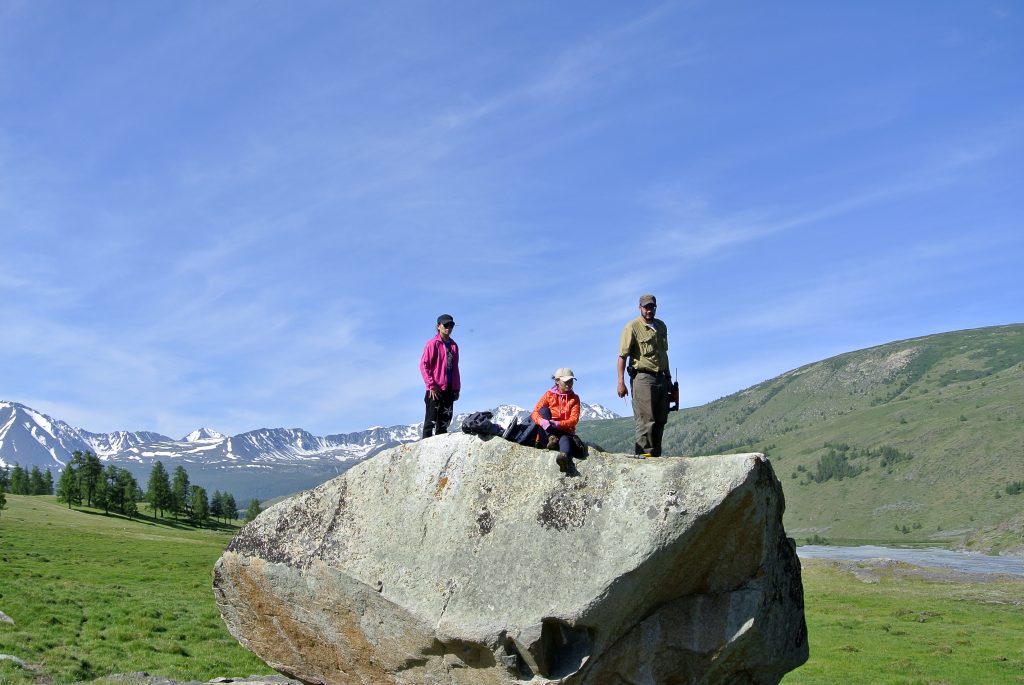
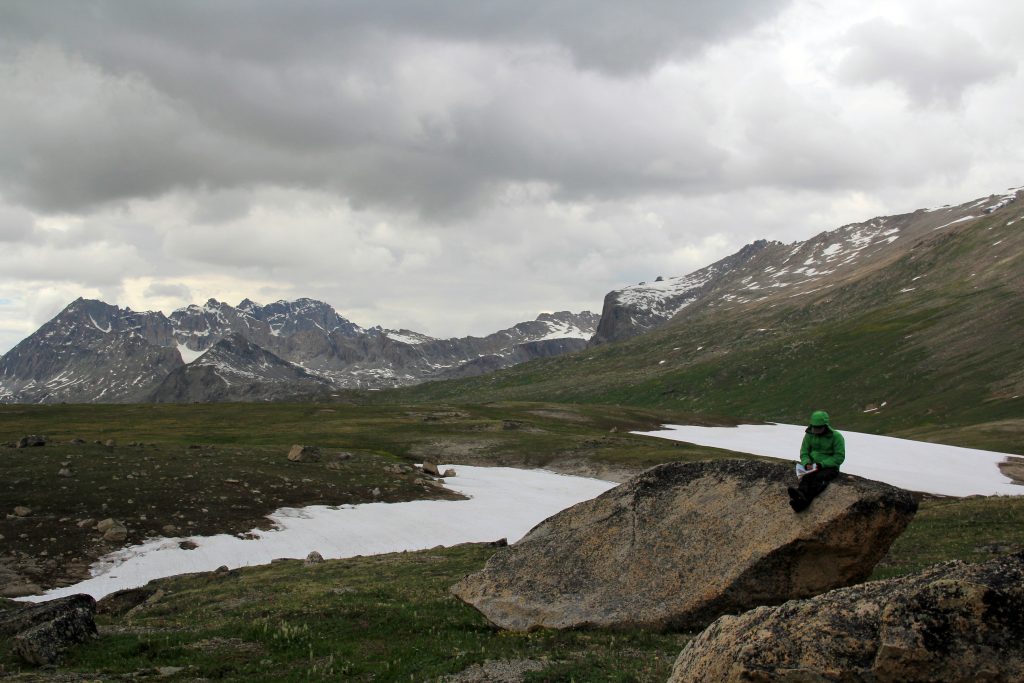
Tanzhuo Liu (“TL”), is an expert in the study of rock varnish. TL will use desert varnish to document periods of changing aridity since the last Ice Age. Richard Kortum, an archeologist and art historian from East Tennessee State University, paid our camp a visit and described for us the most important pieces of rock art in the valley. Richard and TL have been working together with the hopes of dating more precisely the rock art peckings of Iron Age, Bronze Age, Neolithic, and possibly even paleolithic peoples in Mongolia.
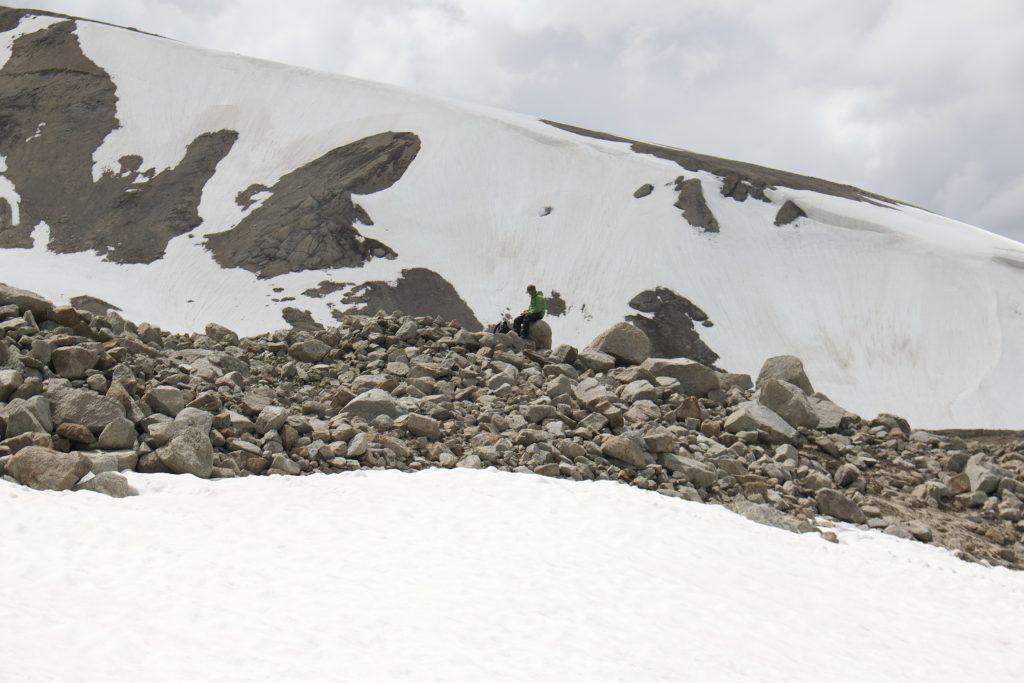
Thank you: We would like to thank everyone at Huvsgol Travel for their help with logistics and permits, without which the trip would not have been possible. Thanks also to our wonderful Mongolian guides, Boldoo, Baagee, Suure, Chackie, and Tomoroo for helping with every aspect of the trip throughout the entire expedition. Thanks to Professor Oyungerel Sambuu and M.U.S.T. for helping with permits and shipping. Thank you to University of Maine, Lamont-Doherty Earth Observatory, University of Maine Presque Isle, and Medill School of Journalism for their support. Thank you to the Comer Science and Education Foundation for their generous financial support. Thank you to all Mongolian and Kazakhs individuals and families who helped us throughout the trip and showed us tremendous hospitality.

Putting the West Antarctic Ice Sheet in context – Dr. George Denton and Dr. Brenda Hall
Funding provided by the National Science Foundation
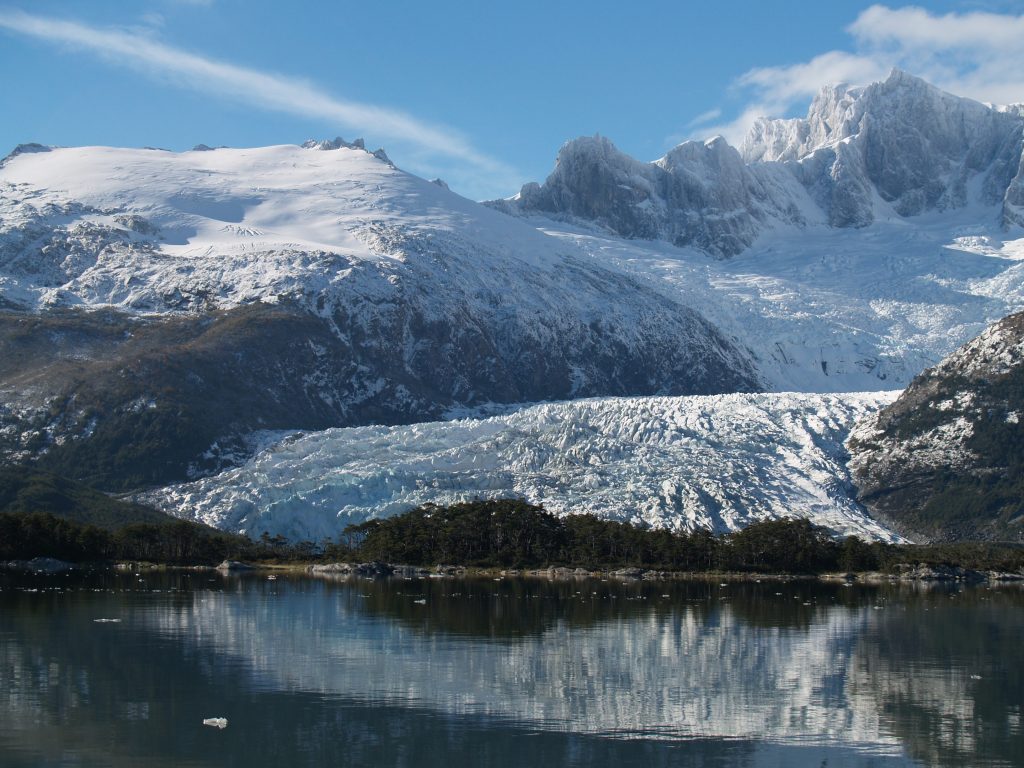
In April, 2012 and 2013, a University of Maine team carried out field work in Cordillera Darwin on Ocean Tramp, captained by Charles Porter. The purpose of the research was to determine the timing of ice retreat from its maximum extent at the Last Ice Age near Bahía Inútil back into the heart of Cordillera Darwin. The overarching question concerned the timing of events during the termination of the last ice age in the Southern Hemisphere and how this relates to mechanisms that cause climate change.
The field team consisted of a number of senior personnel, as well as students, and was divided into two components – a coring group and an exposure-age group. The coring team took samples at the base of bogs to determine the time at which the sites became ice free. The exposure-age group collected samples from rock surfaces for 10Be exposure-age dating, again to determine when sites became free of ice. These complementary methods allow reconstruction of the timing and pace of ice retreat following the last glaciation.

We cored bogs at several sites adjacent to Bahías Ainsworth (Marinelli fjord), Brooks, and Filton on the north side of the range and at Bahía Pía, Caleta Olla, and Ventisquero Holanda on the south . Cores ranged to as much as 11 m in thickness and most penetrated the interglacial sediments to glacial blue clay. Initial dates suggest that the fjords were deglaciated by at least 17,000 years BP (Hall et al., 2013) and that ice was within a kilometer of the present-day glaciers before late-glacial time.
All of the results obtained so far are consistent with rapid deglaciation of the Straits of Magellan and Cordillera Darwin at the end of the last ice age. Comparison of our results and published ages from the maximum ice-sheet extent suggest retreat of more than 100 km in less than 1000 years at the start of the Southern Hemisphere termination. These results are consistent with rapid warming and most likely indicate abrupt southward shift of oceanic fronts.
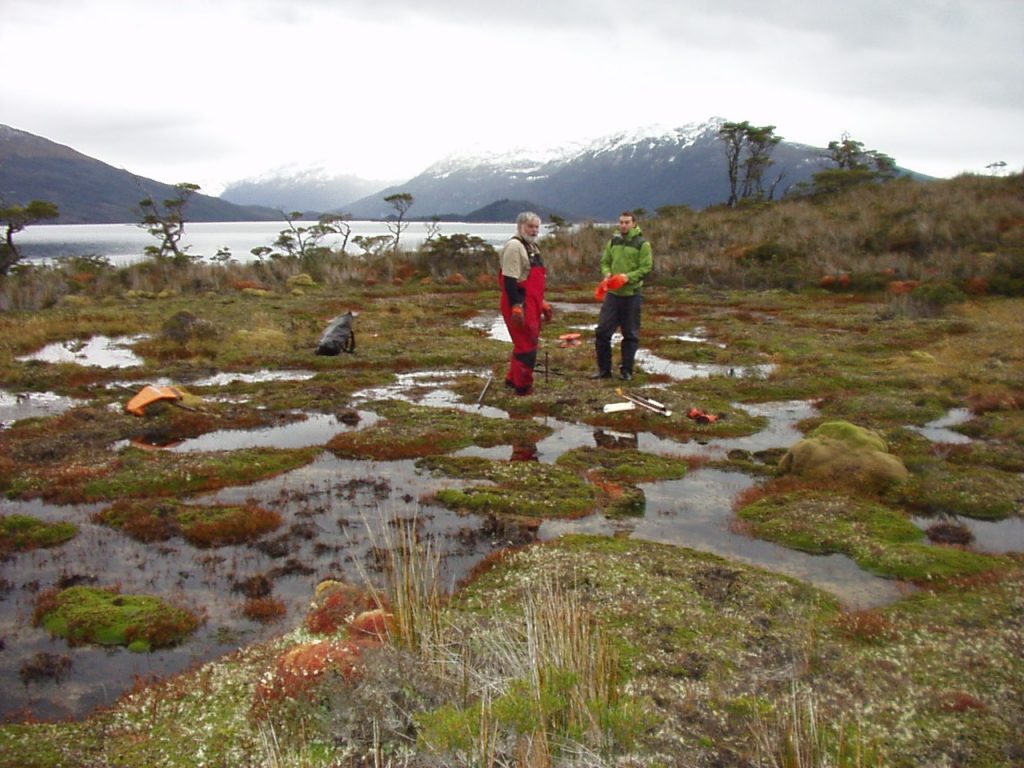
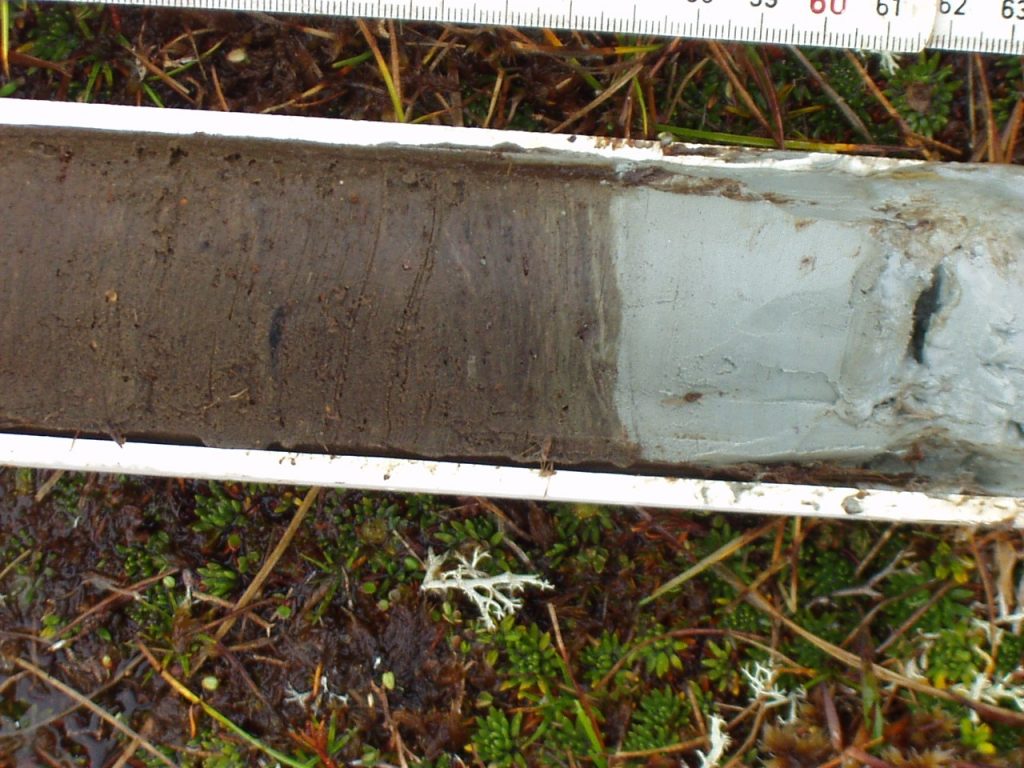
A 10Be chronology of the former Pukaki Glacier, New Zealand: Uncovering the structure of the Last Termination.
Field Team Members: Courtney King, Colin Dowey, Dr. George Denton, Dr. Aaron Putnam (Lamont-Doherty Earth Observatory), and David Barrell (GNS Science, New Zealand)
Funding provided by the National Science Foundation, Comer Science and Education Foundation, and the Quesada Family Foundation
Background: The termination of the last ice age is the largest abrupt climate change of the last 100,000 years, and yet its origin remains enigmatic. Recent work has led to the hypothesis that terminations are characterized by massive outbursts of icebergs and freshwater in the North Atlantic that lead to reduced Atlantic Meridional Overturning Circulation and spread of sea ice (Broecker and Denton, 1990). This northern hemisphere cooling is thought to cause southward shift of the major atmospheric and oceanic fronts, leading to warming in the mid-to-high latitudes of the southern hemisphere (SH) (Denton et al., 2010). Testing this hypothesis and other proposed drivers of ice-age terminations requires detailed and well-dated paleoclimate records through the end of the last ice age. When coupled with glaciological modelling, glacier records yield precise information on changing climate conditions at the periphery of the Southern Ocean during the last termination. For the New Zealand study, we will use glacial geologic mapping and 10Be surface exposure-age dating (SED) to produce a detailed chronology of the onset of the last termination as recorded by the former Pukaki Glacier in the Southern Alps of New Zealand.
With this climate record, we can compare the character of the last termination in New Zealand to the latest Antarctic temperature record from the WAIS-Divide Ice Core, in order to assess the temperature change variance over time at both the northern and southern margins of the Southern Ocean. We will use this comparison to assess the reaction of the Antarctic Ice Sheet to the warming of the last termination (this work is explained in my other two field reports on Hatherton and Darwin Glaciers, Antarctica).
Field work: In January, we traveled from Christchurch to Twizel, a town southwest of Lake Pukaki. From our base in Twizel, we spent a month in the region west of Lake Pukaki mapping the glacial landforms deposited by the former Pukaki Glacier. The mapping provides context for the rock samples we collected for surface exposure-age dating. This region of the Southern Alps has the best-preserved moraine topography on the South Island of New Zealand (Figure 1). The area is familiar to UMaine researchers – many previous graduate students from UMaine have also performed fieldwork along the margins of Lake Pukaki studying various stages of the ice-age cycles. For this field season, we spent a month in the field collecting rock samples along the right lateral moraines deposited by the former Pukaki Glacier (Figures 2 and 3).
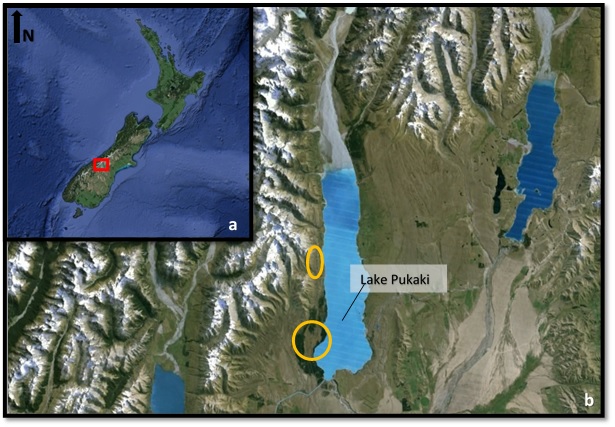
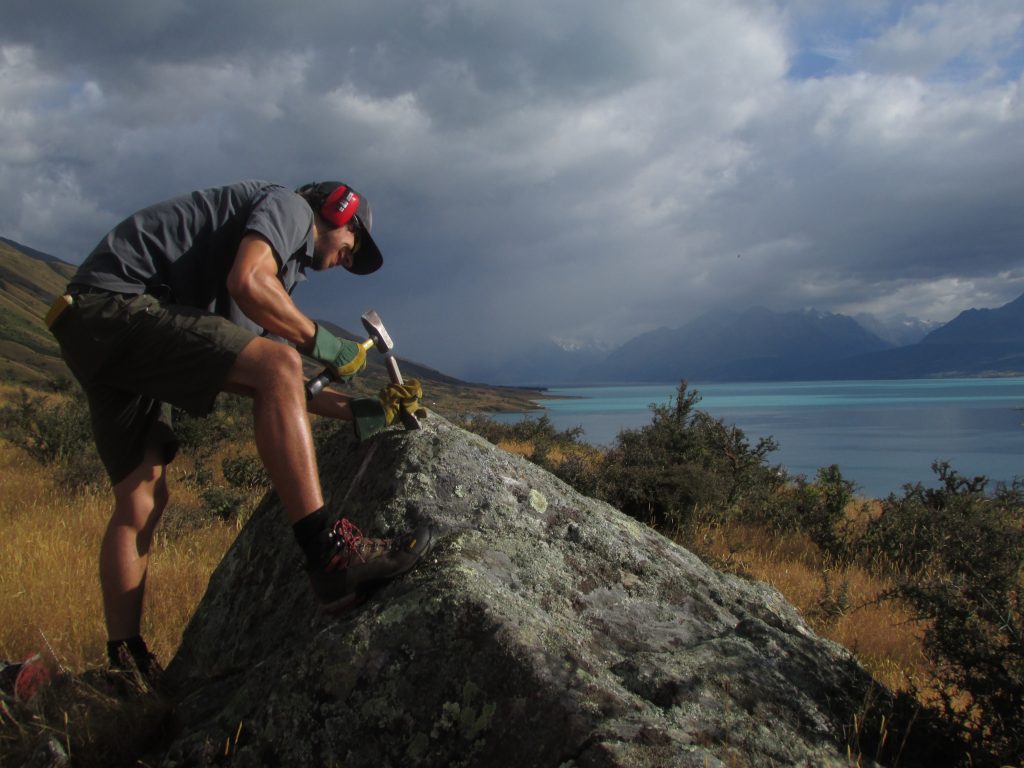
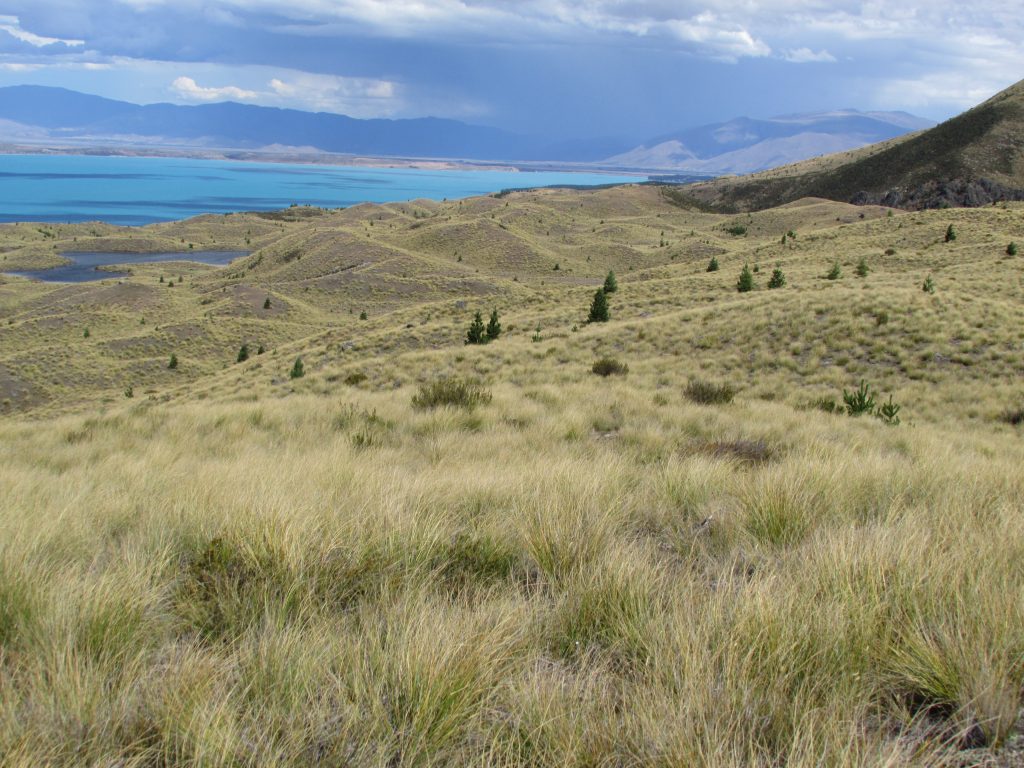
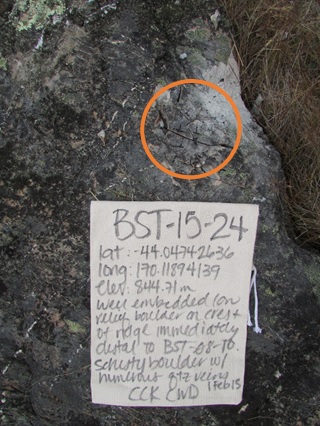
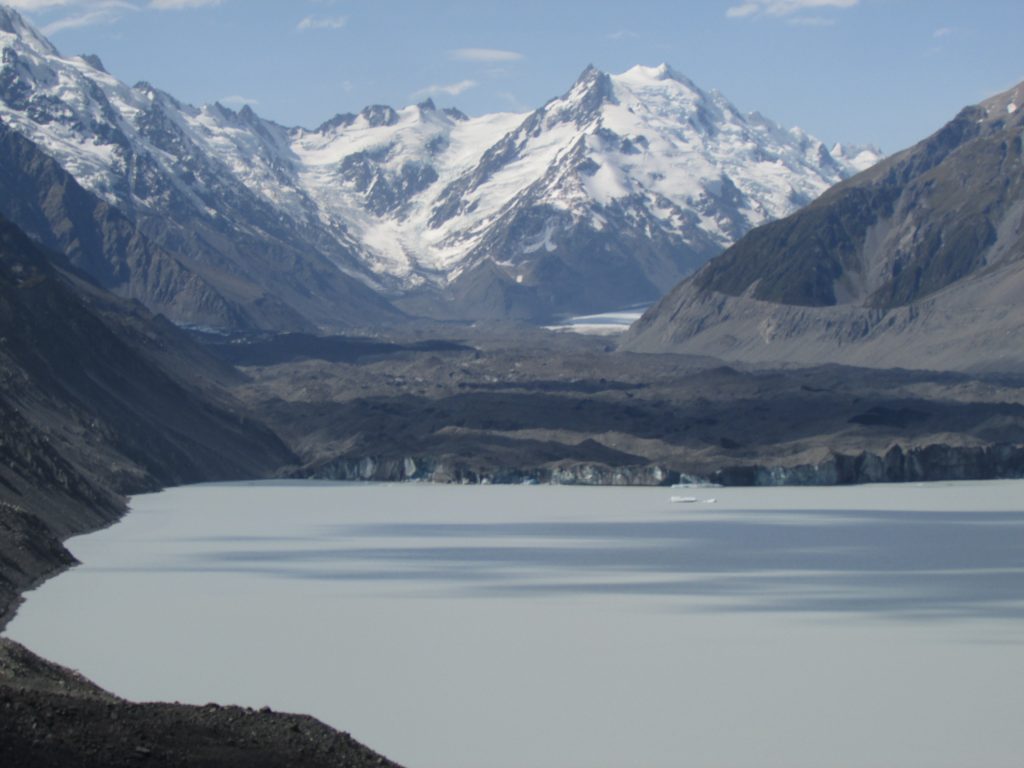
Laboratory work: The rock samples collected for the SED method were sent to the Lamont-Doherty Earth Observatory (LDEO) where they undergo chemical analysis. Surface exposure-age dating is a popular method used to date glacial erratics because it involves counting the number of atoms of 10Be, an isotope of the element Beryllium, which is produced when cosmogenic rays react with quartz (SiO₂) molecules in the rock. As the glacier transports the boulders, erosion removes the outer layer of the rocks, essentially eliminating the accumulated 10Be and resetting the clock that measures how long it’s been exposed to the cosmic rays. The clock begins once the erratics are deposited by the glacial ice because they begin accumulating the 10Be isotope. Chemically processing a sample-set takes several months. Once the 10Be is isolated, the samples are analyzed at the Center for Accelerator Mass Spectrometry facility at Lawrence Livermore National Laboratory.
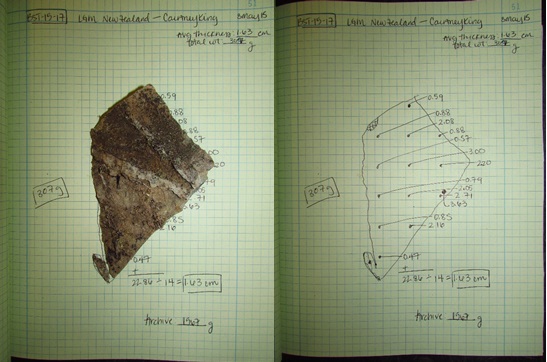
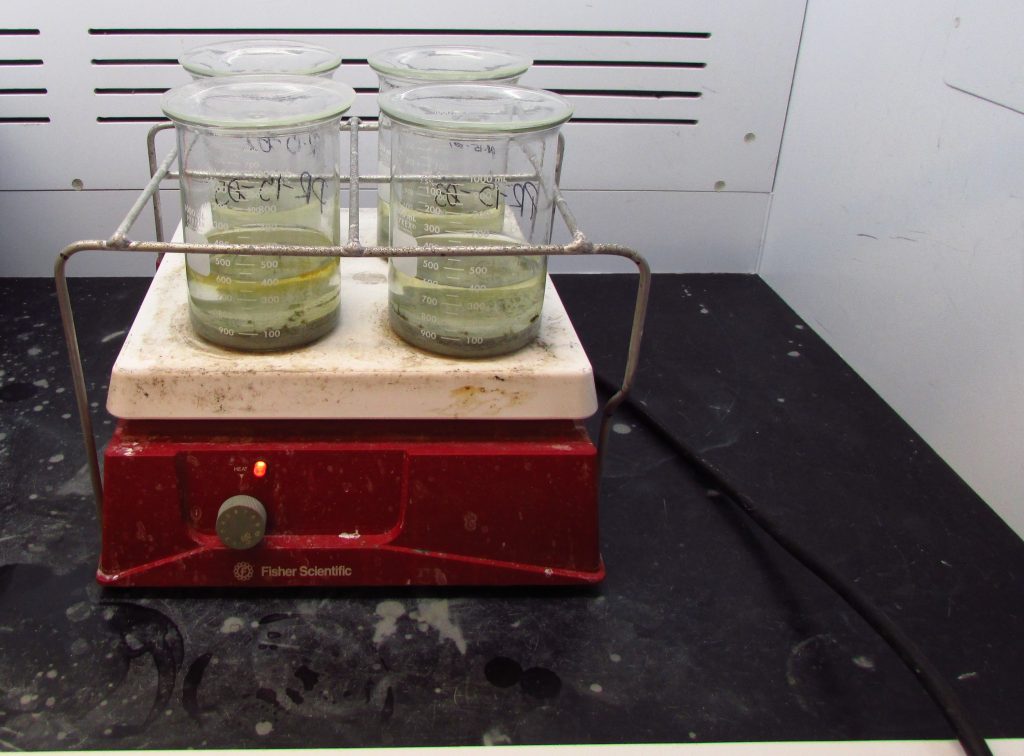
Future work: In addition to the rock sample analyses, we will digitize the field maps using ArcGIS software to add to the compilation of geomorphologic maps produced for this region (eg. Barrell et al., 2013; Doughty et al., 2015, 2013; Kaplan et al., 2013; Putnam et al., 2012, 2010a, 2010b).
References:
Barrell, D.J.A., Anderson, B.G., Denton, G.H., 2011. Glacial Geomorphology of the central South Island, New Zealand. GNS Science Monograph 27. Lower Hutt, GNS Science. 1 map (5 sheets) + 81p.
Broecker, W.S., Denton, G.H., 1990. The Role of Ocean-Atmosphere reorganizations in glacial cycles. Quat. Sci. Rev. 9, 305–341.
Denton, G.H., Anderson, R.F., Toggweiler, J.R., Edwards, R.L., Schaefer, J.M., Putnam, a E., 2010. The last glacial termination. Science 328, 1652–1656. doi:10.1126/science.1184119
Doughty, A.M., Anderson, B.M., Mackintosh, A.N., Kaplan, M.R., Vandergoes, M.J., Barrell, D.J. a., Denton, G.H., Schaefer, J.M., Chinn, T.J.H., Putnam, A.E., 2013. Evaluation of Lateglacial temperatures in the Southern Alps of New Zealand based on glacier modelling at Irishman Stream, Ben Ohau Range. Quat. Sci. Rev. 74, 160–169. doi:10.1016/j.quascirev.2012.09.013
Doughty, A.M., Schaefer, J.M., Putnam, A.E., Denton, G.H., Kaplan, M.R., Barrell, D.J.A., Andersen, B.G., Kelley, S.E., Finkel, R.C., Schwartz, R., 2015. Mismatch of glacier extent and summer insolation in Southern Hemisphere mid-latitudes. Geology 43, 407–410. doi:10.1130/G36477.1
Kaplan, M.R., Schaefer, J.M., Denton, G.H., Doughty, A.M., Barrell, D.J.A., Chinn, T.J.H., Putnam, A.E., Andersen, B.G., Mackintosh, A., Finkel, R.C., Schwartz, R., Anderson, B., 2013. The anatomy of long-term warming since 15 ka in New Zealand based on net glacier snowline rise. Geology 41, 887–890. doi:10.1130/G34288.1
Putnam, A.E., Denton, G.H., Schaefer, J.M., Barrell, D.J. a., Andersen, B.G., Finkel, R.C., Schwartz, R., Doughty, A.M., Kaplan, M.R., Schlüchter, C., 2010a. Glacier advance in southern middle-latitudes during the Antarctic Cold Reversal. Nat. Geosci. 3, 700–704. doi:10.1038/ngeo962
Putnam, A.E., Schaefer, J.M., Barrell, D.J.A., Vandergoes, M., Denton, G.H., Kaplan, M.R., Finkel, R.C., Schwartz, R., Goehring, B.M.M., Kelley, S.E., 2010b. In situ cosmogenic 10Be production-rate calibration from the Southern Alps, New Zealand. Quat. Geochronol. 5, 392–409. doi:10.1016/j.quageo.2009.12.001
Putnam, A.E., Schaefer, J.M., Denton, G.H., Barrell, D.J. a., Finkel, R.C., Andersen, B.G., Schwartz, R., Chinn, T.J.H., Doughty, A.M., 2012. Regional climate control of glaciers in New Zealand and Europe during the pre-industrial Holocene. Nat. Geosci. 5, 627–630. doi:10.1038/ngeo1548
Vandergoes, M.J., Dieffenbacher-Krall, A.C., Newnham, R.M., Denton, G.H., Blaauw, M., 2008. Cooling and changing seasonality in the Southern Alps, New Zealand during the Antarctic Cold Reversal. Quat. Sci. Rev. 27, 589–601. doi:10.1016/j.quascirev.2007.11.015
History of grounded ice in the Ross embayment since the Last Glacial Maximum using the glacial geology alongside the Hatherton and Darwin glacier system, Antarctica – Courtney King, Brenda Hall, John Stone, Trevor Hillebrand
[2013-present]
Field Team Members: Courtney King, Dr. Brenda Hall, Dr. John Stone (University of Washington), and Trevor Hillebrand (UW)
Funding provided by the National Science Foundation
Introduction: The future of the Antarctic Ice Sheet (AIS) is of great concern because if it completely melted, sea-level would rise greater than 60 m. In particular, the marine-based West Antarctic Ice Sheet is thought to be inherently unstable and susceptible to rapid collapse (Hughes, 1973; Mercer, 1978). In order to better understand the stability of the AIS and its potential for future collapse, we need to gain insight into its past. During the last glacial maximum, the most recent time at which the AIS was at its greatest extent (approximately 20,000 years ago), ice grounded in the Ross embayment. This grounded ice buttressed inflowing outlet glaciers from the East Antarctic Ice Sheet, causing them to thicken and flow into currently ice-free valleys of the Transantarctic Mountains (Bockheim et al., 1989; Denton et al., 1989; Hall et al., 2013). Obtaining a chronology using the glacial geology deposited by the formerly thicker ice will provide insight into not only the timing of the last glacial maximum, but an ice-thinning history of the AIS. Along with our collaborators from the University of Washington, Dr. John Stone and Trevor Hillebrand, my advisor, Dr. Brenda Hall, and I spent four weeks collecting rock samples for surface exposure-age dating and algae samples for radiocarbon dating that will produce precise ice-thinning chronologies from alongside Hatherton Glacier, Antarctica.
Background: This year’s field expedition is a continuation of last year’s work along Hatherton Glacier (Figure 1). Our goal for this project is to determine the timing of the last glacial maximum (LGM) and subsequent recession for the Antarctic Ice Sheet (AIS). Limited radiocarbon ages from alongside the Hatherton Darwin glacier system support the hypothesis for grounded ice in the Ross embayment during the LGM (Bockheim et al., 1989). In contrast, two recent publications presented surface exposure-ages from the Lake Wellman area and the region alongside upper Hatherton Glacier, in which authors postulate a much different history for grounded ice in the Ross embayment during the last glacial period (Joy et al., 2014; Storey et al., 2010). In the Lake Wellman area, Storey et al. (2010), suggests there was little-to-no change in glacier extent, and alongside upper Hatherton Glacier, Joy et al. (2014) concludes that due to the lack of surface exposure-age dates covering the LGM period, the ice extent during the last glacial period was likely lower than present. In order to test these hypotheses, we want to construct precise radiocarbon and surface exposure-age chronologies from numerous sites along the longitudinal profile of the Hatherton Darwin glacier system.
Field Work: Last year, we visited both the Lake Wellman area and the section of valleys alongside upper Hatherton Glacier, and for this year’s field season we chose a site located between those two, Magnis Valley. In addition, we went to Diamond Hill, located near the mouth of Darwin Glacier, a major outlet glacier that flows through the Transantarctic Mountains from East Antarctica (Figure 1). Dr. Hall and I collected fossil algae samples along transects perpendicular to the present ice margin that will be radiocarbon dated. Meanwhile, Dr. Stone and graduate student, Trevor Hillebrand, collected rock samples for surface exposure-age dating. Both dating methods will allow us to reconstruct a longitudinal profile of ice extent during the LGM, and track the subsequent ice recession.
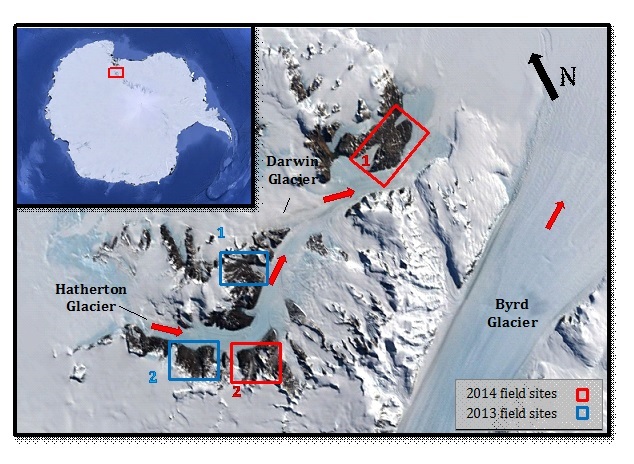
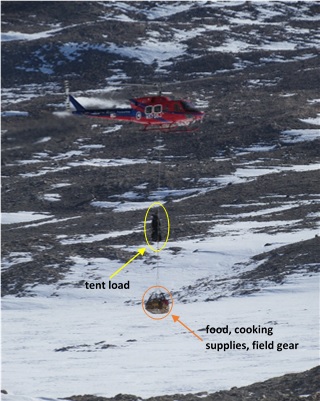
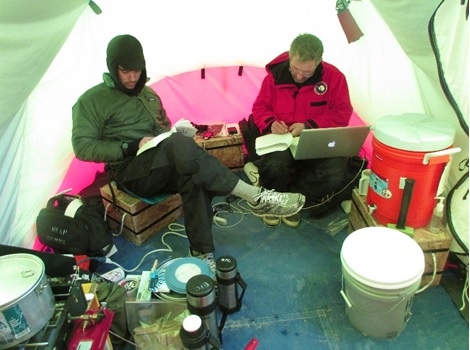
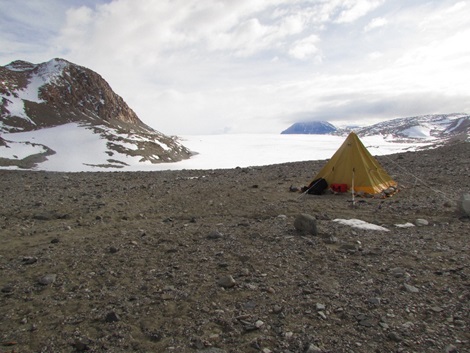
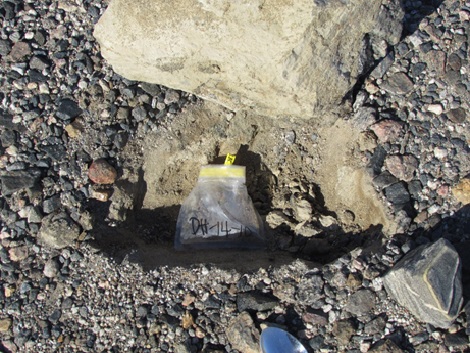
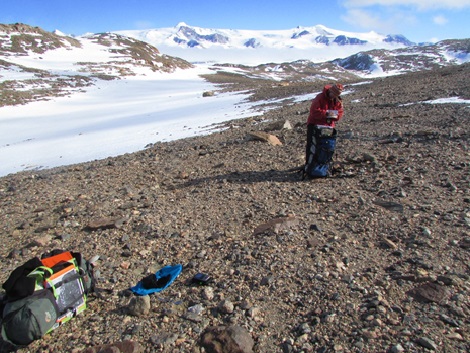
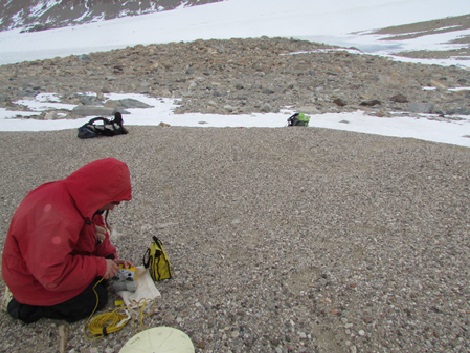

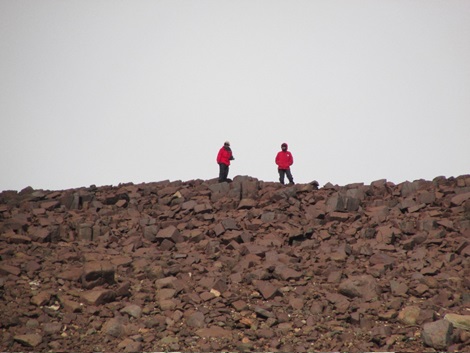
Continuing work (mapping and dating): I began working on surficial geomorphic maps of the two field sites we visited last year, and this year I will also digitize the field maps of Magnis Valley and Diamond Hill using ArcGIS software. The geomorphic maps will help us develop a longitudinal profile of the Hatherton Darwin glacier system ice extent for the LGM and subsequent recession. We have also previously sent and, from this year’s collection, will send more fossil algae samples to the Woods Hole Oceanographic Institute for radiocarbon dating. Our collaborators from the University of Washington, Dr. John Stone and Trevor Hillebrand, collected rock samples (predominately sandstone) for cosmogenic surface exposure-age dating using 10-Beryillium (10Be). This is a popular method used to date glacial erratics because it involves counting the number of atoms of 10Be, an isotope of the element Beryllium, which is produced when cosmogenic rays react with quartz (SiO₂) molecules on the rock’s surface. As erratics are transported in the ice, erosion removes the outer layer of the rocks, essentially eliminating the accumulated 10Be and resetting the clock that measures how long it’s been exposed to cosmic rays. The clock begins once the erratics are deposited by the glacial ice and exposed to cosmic rays because they begin accumulating the 10Be isotope. After the rocks samples undergo chemical analyses for the 10Be at the University of Washington’s Cosmogenic Nuclide Laboratory, they will then be sent to Lawrence-Livermore National Laboratory where the Accelerator Mass Spectrometry process is used to count the number of Beryllium isotopes for each sample, which in turn, allows us to calculate an age for the samples. Radiocarbon and surface exposure-age dating techniques are tools that will allow us to assign ages to the drift deposits we mapped in the field, leading us to hypothesize the history and stability of the AIS.
References:
Bockheim, J.G., Wilson, S.C., Denton, G.H., Andersen, B.G., Stuiver, M., 1989. Late Quaternary ice-surface fluctuations of Hatherton Glacier, Transantarctic Mountains. Quat. Res. 31, 229–254. doi:10.1016/0033-5894(89)90007-0
Denton, G.H., Bockheim, J.G., Wilson, S.C., Stuiver, M., 1989. Late Wisconsin and early Holocene glacial history, inner Ross embayment, Antarctica. Quat. Res. 182, 151–182.
Hall, B.L., Denton, G.H., Stone, J.O., Conway, H., 2013. History of the grounded ice sheet in the Ross Sea sector of Antarctica during the Last Glacial Maximum and the last termination. Geol. Soc. London, Spec. Publ. 381, 167–181. doi:10.1144/SP381.5
Hughes, T., 1973. An unstable tetrahedral mantle-convection model, continental drift, and polar ice sheets. Tectonophysics 17, 73–88.
Joy, K., Fink, D., Storey, B., Atkins, C., 2014. A 2 million year glacial chronology of the Hatherton Glacier, Antarctica and implications for the size of the East Antarctic Ice Sheet at the last glacial maximum. Quat. Sci. Rev. 83, 46–57. doi:10.1016/j.quascirev.2013.10.028
Storey, B.C., Fink, D., Hood, D., Joy, K., Shulmeister, J., Riger-Kusk, M., Stevens, M.I., 2010. Cosmogenic nuclide exposure age constraints on the glacial history of the Lake Wellman area, Darwin Mountains, Antarctica. Antarct. Sci. 22, 603–618. doi:10.1017/S0954102010000799
Reconstructing Late Quaternary climate variability in the tropical Andes – Gordon Bromley, Brenda Hall, Kurt Rademaker
[2006-present]
Field Team Members: Gordon Bromley, Kurt Rademaker, Peter Strand, Ben Gross, Matt Kohler, Claire Todd (Pacific Lutheran University), Matthew Hegland (PLU), Matthew Schmitt (PLU), Brian Donahue (PLU), Kim Martineau (Lamont-Doherty).
Funding provided by Dan & Betty Churchill and the National Science Foundation
This ongoing investigation, which began in 2006, is utilising the geologic record of glaciation preserved in the southern Peruvian Andes in order to improve our understanding of past climate variability in the tropics. In contrast to higher latitudes, the tropical climate record is virtually unknown and outstanding questions remain, including the precise timing of the last glacial maximum (LGM), the magnitude of LGM cooling in the tropics, and the occurrence of abrupt climate events (such as the Younger Dryas and Antarctic Cold Reversal) during the last glacial termination. Even less is known of how the steep precipitation gradients inherent to the Andes might have influenced the tropical response to these important events. Why are such questions important? Because the tropics are the primary source of both heat and water vapour for the globe, because they are home to over a third of the world’s population, and because they offer unparalleled insight into the causes and mechanisms of global climate variability.
We are exploiting the abundance of moraines preserved at sites in both the humid and arid Peruvian Andes to reconstruct both the timing and magnitude of Late Quaternary climate changes. These landforms record past periods when glaciers were more extensive than today and form the basis for palaeo-snowline reconstruction and glacial chronology, using surface-exposure dating. At Nevado Coropuna, located in the arid Cordillera Occidental, we are employing cosmogenic 3He to date andesitic erratics deposited during and since the LGM. Helium samples are being run at Lamont-Doherty Earth Observatory. Meanwhile, at Laguna Aricoma, located in the relatively humid Cordillera Oriental, we are using cosmogenic 10Be to date quartzite and granite surfaces in glacial contexts. Beryllium samples are prepared at Lamont-Doherty Earth Observatory. While these chronologies are works in progress, our results already afford valuable insights into patterns of glaciation – and therefore climate variability – from the depths of the last ice age to the present interglacial (Bromley et al., 2009, 2011a, 2011b). Moreover, we have documented considerable differences in the magnitude of snowline lowering at the LGM between arid and humid sites. A key challenge now is to determine whether this pattern relates to climate or to other factors, such as topography. A further challenge is to determine whether the tropics behave as a single entity or as separate hemispheres, as this has implications for the range of influence of the tropics. Thus, we are expanding our research into the northern Andes of Colombia in conjunction with collaborators at the Escuela de Ingeniería de Antioquia, Medellín, and Berkeley Geochronology Center.
To learn more about this project, and associated archaeologic investigations in Peru, follow this link to a short video prepared in 2013 by Kim Martineau of Lamont-Doherty Earth Observatory: http://www.ldeo.columbia.edu/news-events/climate-peruvian-andes-early-humans-modern-challenges. Additionally, we maintained a research blog of our 2011 field season that was archived as part of Columbia Earth Institute’s “State of the Planet” series (http://blogs.ei.columbia.edu/tag/peru-glaciers/).
Publications:
Bromley, G.R.M., Winckler, G., Schaefer, J.M., Kaplan, M., Licht, K.J., Hall, B.L., 2014. Pyroxene separation by HF leaching and its impact on helium surface-exposure dating. Quaternary Geochronology 23, 1-8.[http://www.sciencedirect.com/science/article/pii/S1871101414000351]
Audio presentation with slides: http://audioslides.elsevier.com/viewerlarge.aspx?doi=10.1016/j.quageo.2014.04.003&Source=0&resumeTime=4086.7120181405894&resumeSlideIndex=2
Bromley, G.R.M., Hall, B.L., Rademaker, K.M., Todd, C.E., Racoviteanu, A.E., 2011. Late Pleistocene snowline fluctuations at Nevado Coropuna (15?S), southern Peruvian Andes. Journal of Quaternary Science 26, 305-317. [http://onlinelibrary.wiley.com/doi/10.1002/jqs.1455/abstract]
Bromley, G.R.M., Hall, B.L., Schaefer, J.M., Winckler, G., Todd, C.E., Rademaker, K.M., 2011. Glacier fluctuations in the southern Peruvian Andes during the late-glacial period, constrained with cosmogenic 3He. Journal of Quaternary Science 26, 37-46. [http://onlinelibrary.wiley.com/doi/10.1002/jqs.1424/abstract]
Bromley, G.R.M., Schaefer, J.M., Winckler, G., Hall, B.L., Todd, C.E., Rademaker, K.M., 2009. Relative timing of last glacial maximum and late glacial events in the tropical Andes. Quaternary Science Reviews 28, 2514-2526. [http://www.sciencedirect.com/science/article/pii/S0277379109001711]
Chronology of the Loch Lomond Advance in Scotland – Gordon Bromley, Aaron Putnam, Kurt Rademaker
[2010-present]
Field Team Members: Gordon Bromley, Aaron Putnam, Kurt Rademaker.
Funding provided by the Dan & Betty Churchill, the Lamont Climate Center, and UMaine.
This research aims to decipher the full manifestation of abrupt climate change in the circum North Atlantic. Our objective is to place firm chronologic constraint on the last pulse of glaciation in the British Isles – the Loch Lomond Advance. Being located on the margin of the North Atlantic Ocean, former glaciers in the Scottish Highlands will have been highly sensitive to changes in upwind sea-surface temperature and circulation changes. Thus, the Scottish moraine record is an unparalleled tool for deciphering the terrestrial impact of North Atlantic ‘stadial’ events such as the Younger Dryas and Heinrich Stadial 1. Specifically, we are working to ascertain exactly how the cryosphere responded to the extreme seasonality of these events. As a first step, we focused on collecting and dating basal sediments overlying Loch Lomond tills at Rannoch Moor – the former centre of the Loch Lomond ice cap – in order to provide a robust minimum age for the end of the event. Our approach incorporated detailed field mapping and sediment coring, using a Livingstone corer, to reach the earliest post-glacial sediments on Rannoch Moor. Plant macrofossils extracted from the basal sections of the cores have been radiocarbon dated to provide the minimum-limiting chronology for deglaciation. Our findings to date, published recently in Proceedings of the National Academy of Sciences (Bromley et al., 2014), suggest the Younger Dryas – and by extension other stadial events – was characterised by summertime warming in the North Atlantic, resulting in glacier retreat, rather than uniform cooling. Following on from that work, we are now applying cosmogenic 10Be surface-exposure dating to late-glacial moraines in the NW Highlands in order to refine the glacial chronology and resolve directly the timing of this late-glacial event, from its maximum extent through deglaciation. All 10Be samples are being run through the UMaine geochronology lab.
To learn more about Gordon and Kurt’s collaboration with the Scottish Land Estates and the Dundonnell and Eilean Darrach Estates, click here.
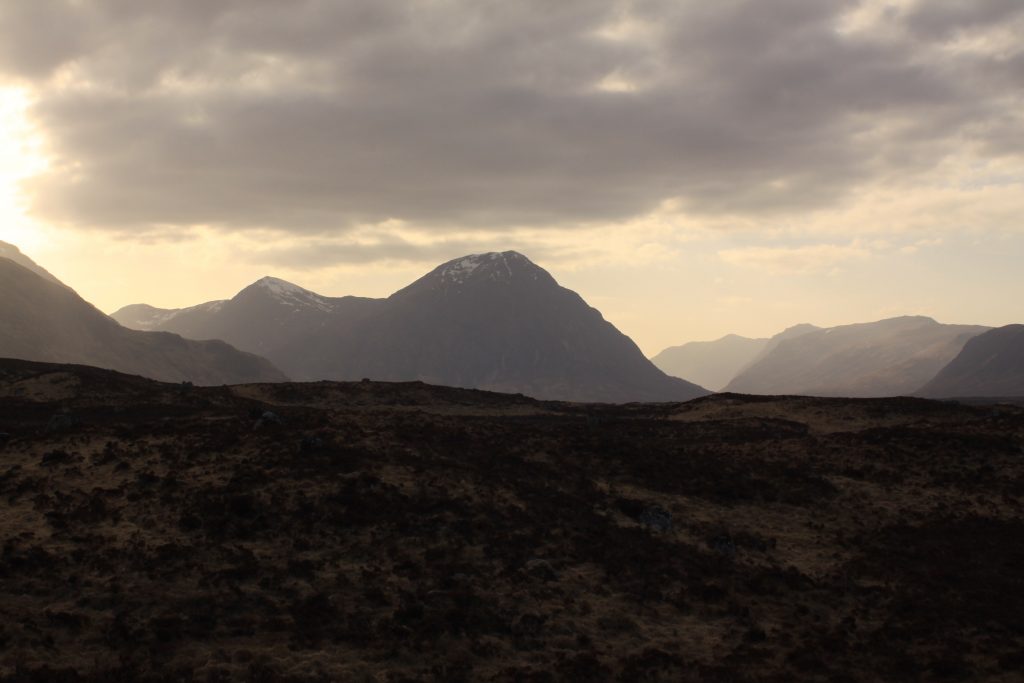
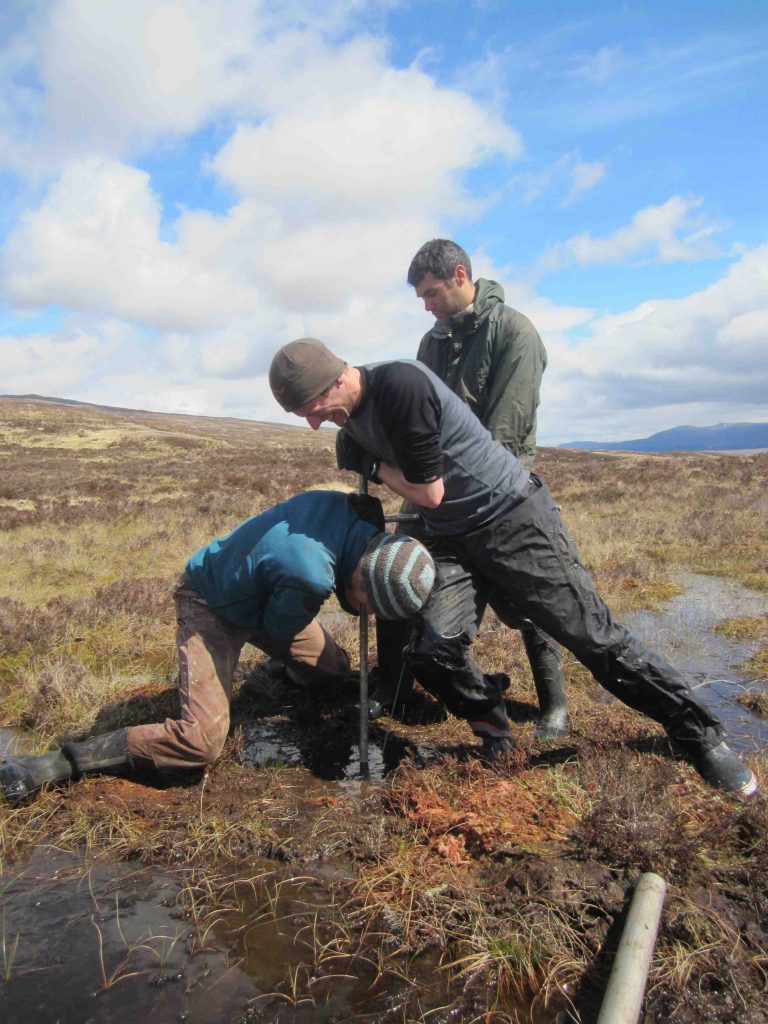
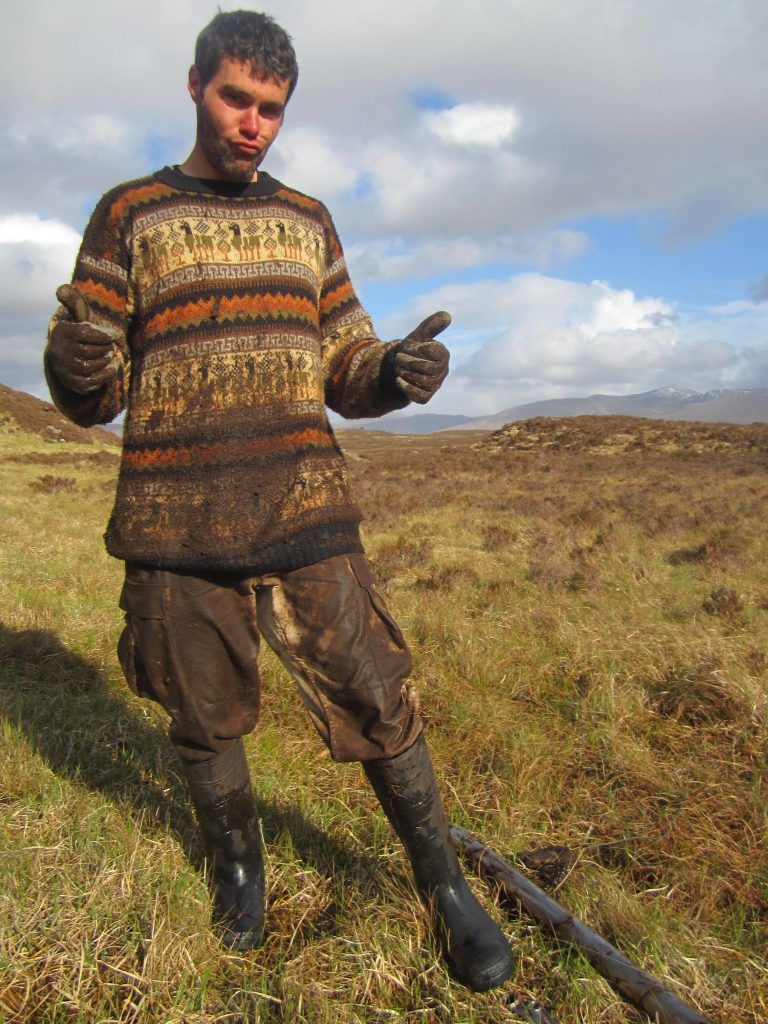
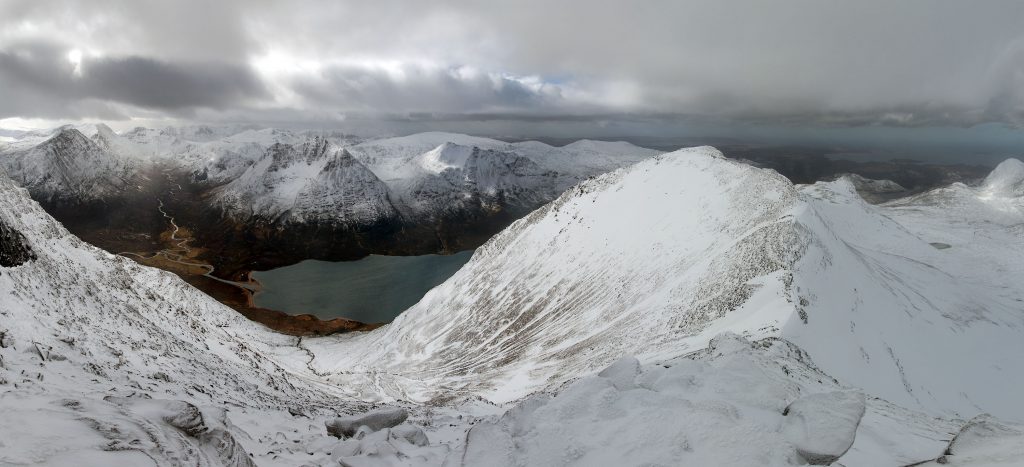
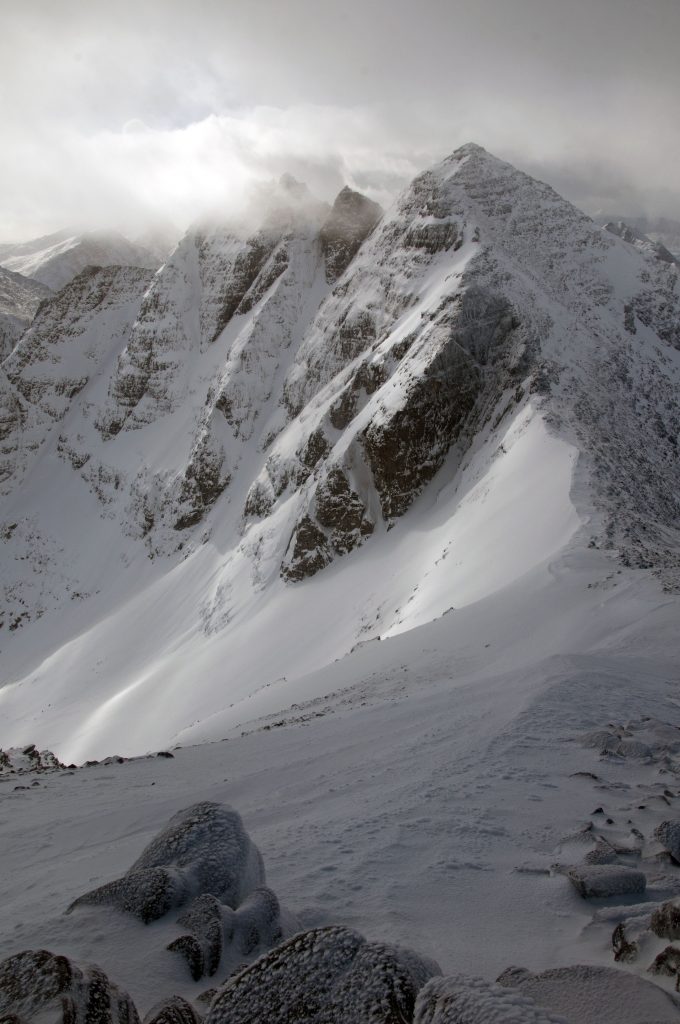
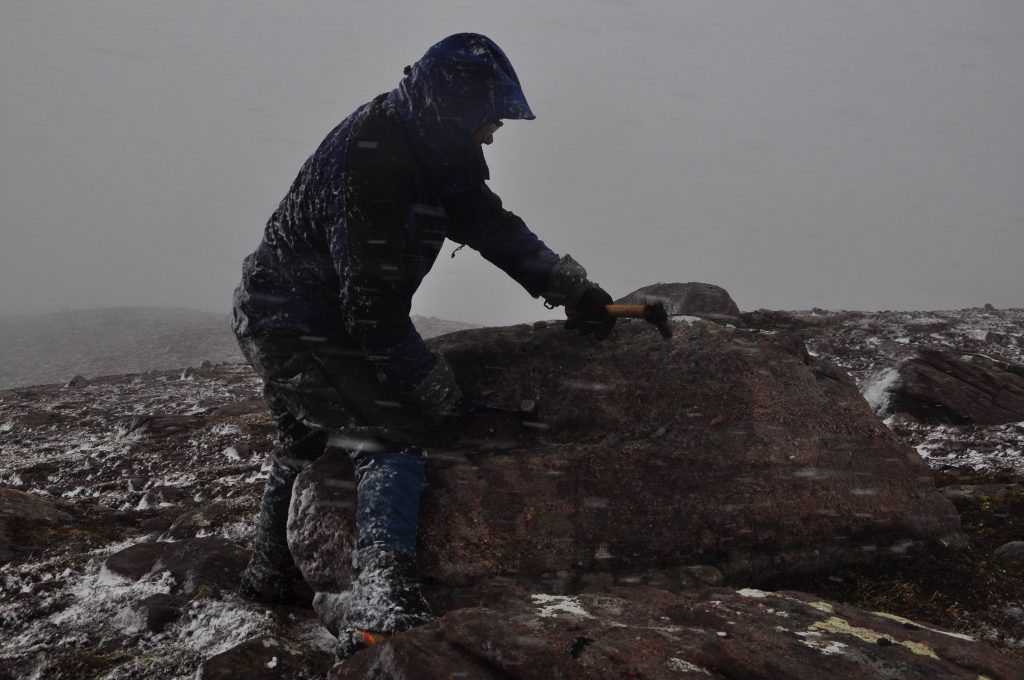
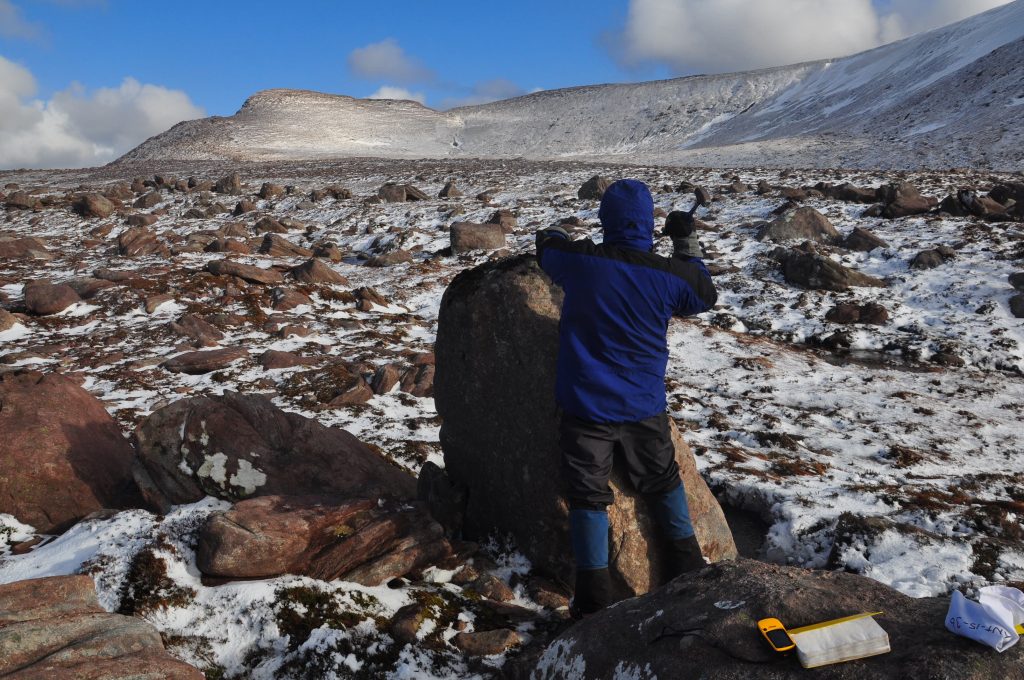
Publications:
Gordon R. M. Bromley, Aaron E. Putnam, Kurt M. Rademaker, Thomas V. Lowell, Joerg M. Schaefer, Brenda Hall, Gisela Winckler, Sean Birkel, Harold W. Borns, 2014. Younger Dryas deglaciation of Scotland driven by warming summers. PNAS 111, 6215-6219. www.pnas.org/cgi/doi/10.1073/pnas.1321122111.
Terminal Pleistocene glaciation of high-altitude Andes near Chivay, Peru: Implications for tropical paleoclimate and Paleoindian-age people – Scott Braddock, Paul Pluta
[2013-present]
Field Team Members: Scott Braddock, Gordon Bromley, Paul Pluta
The tropical Andes are an ideal location to study past abrupt climate change and the impacts such events imposed on Paleoindian populations. Previous work by Sandweiss et al. (1998) in southern Peru suggested the glacial history of this region influenced when the Chivay source of obsidian became available to early populations. While continuing to determine the pattern of southern Peru’s late Pleistocene climate, we also can help answer whether glacial fluctuations made certain important resources inaccessible during this period.
Our proposed research aims to use glacial geology to address how glaciers behaved during the Terminal Pleistocene in the tropical Andes and how the impacts of ice-marginal fluctuations may have influenced human-environment interactions in southern Peru. To address these questions we propose to determine: (1) whether glacial features in the Chivay region date to the Younger Dryas, or show a different signal correlated to the Antarctic Cold Reversal, and (2) if the Chivay obsidian source was covered by a glacial readvance at the end of the Pleistocene, making the site inaccessible to early South Americans, such as the occupants of Quebrada Jaguay.
Field work was completed this summer during July. The team successfully collected samples from glacial erratics that will be used to determine when local glaciers had receded and exposed the Chivay source of obsidian.
Funding for this field expedition provided by Dan and Betty Churchill Exploration Fund.
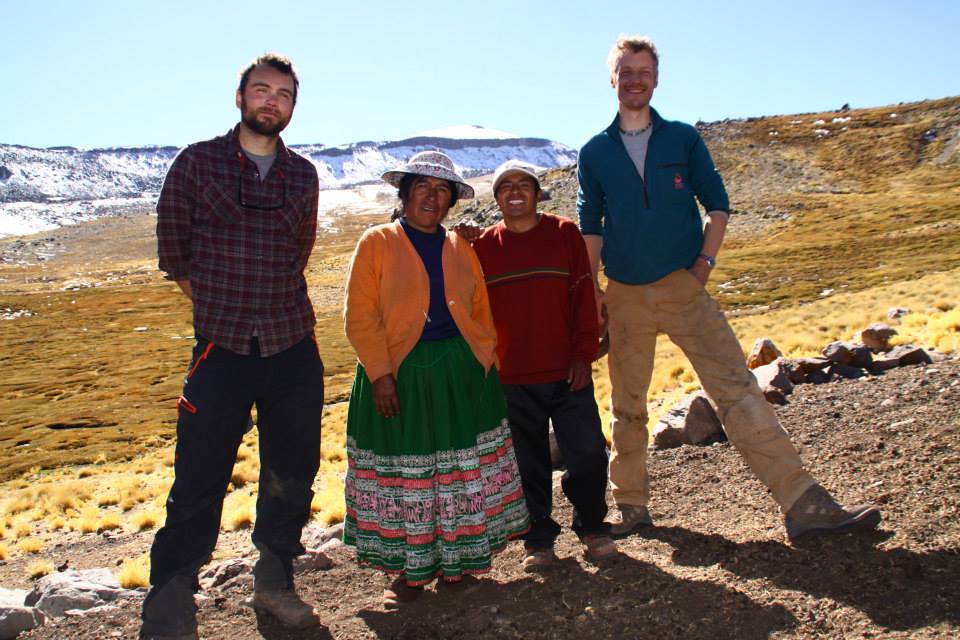
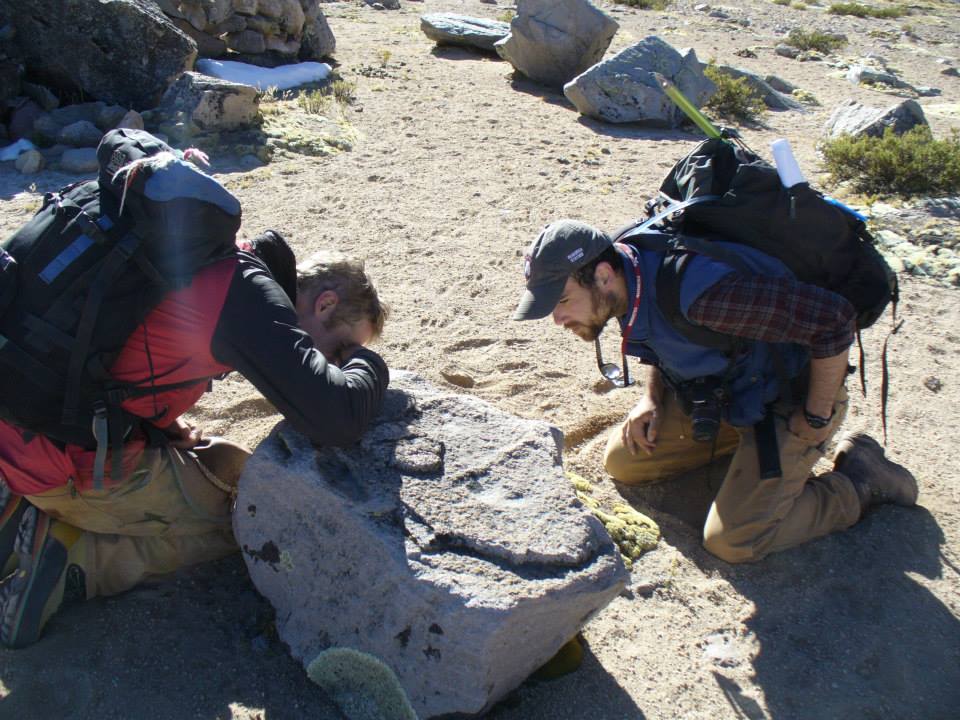
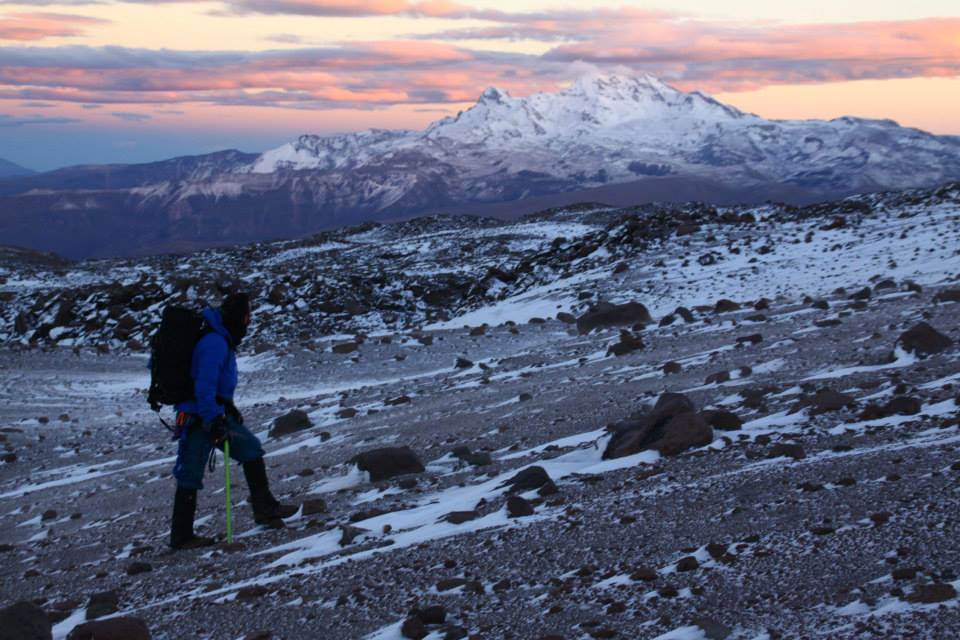
Dating the LGM in Tierra del Fuego – Jennifer Lennon, Brenda Hall
[2012-present]
Field Team Members: Brenda Hall, Alex Introne, Mike Kaplan, Jen Lennon, Tom Lowell, Aaron Putnam
Chilean Tierra del Fuego, March 25, 2013- April 2, 2013
Funding provided by Dan and Betty Churchill, National Science Foundation
Introduction: One implication of Milankovitch’s orbital theory of ice ages is that glacial cycles should be asynchronous between the northern and southern mid-latitudes, because insolation forcing is out of phase between the two hemispheres. However, evidence at present points to a near-synchronous termination (a rapid transition from glacial to interglacial conditions) at the end of the last glacial maximum (LGM) in both hemispheres. One way to test hypotheses for the ice ages and their terminations is to create detailed glacial chronologies that record the timing of past ice fluctuations. Globally distributed records allow one to make geographic comparisons to isolate potential mechanisms that drive ice ages.
The glacial history of southernmost South America is not well-documented at present, but is important for understanding the characteristics of the Southern Hemisphere ice age and termination. During March and April of 2013, a field team from the University of Maine (along with colleagues from Lamont-Doherty Earth Observatory and the University of Cincinnati) traveled to Bahía Inútil, Chile, to collect data to improve the local glacial record, and that allow for the testing of larger hypotheses concerning the termination of the last ice age. The field work consisted of glacial mapping and the collection of rock samples from boulder surfaces for surface-exposure age dating. This technique results in precise dates of glacial landform construction. When a quartz-bearing boulder melts out of a receding ice lobe, the boulder surface is exposed to cosmogenic rays. When these rays strike the surface, spallation reactions occur within the quartz and produce 10Be atoms at known production rates.
Location and Setting: The Bahía Inútil field area lies at 53°S and 69ºW on the equatorial side of the Antarctic Frontal Zone. The Straights of Magellan and Bahía Inútil are large marine inlets surrounded on all sides by low elevation coastal areas. During the LGM (~26,000 -18,000 years before present) ice flowed through the Straits of Magellan from mountains to the west and south, such as Cordillera Darwin. The moraines adjacent to Bahía Inútil represent the fluctuations of a major ice lobe during the LGM until the start of the last termination. The moraines examined during this field season lie just to the south of Bahía Inútil, on the Chilean side of Tierra del Fuego. The landscape is covered with heath-like vegetation and few trees. The moraines here are subtle, appearing as boulder strings on low, curving ridges. The windward surfaces of the boulders are very weathered.
Field Season: The field party arrived in Punta Arenas, Chile, on the 25th of March, and by the next day, we had 4×4 vehicles loaded to the brim with field team members and equipment. To get to the field site, we first took a ferry across the Straits of Magellan. We drove south through Tierra del Fuego until arrival in Bahía Inútil. Most of Tierra del Fuego is privately owned, and the field work completed during this expedition was done on two large estancias, Estancia Tres Hermanos, and Estancia Rosa Irene, with landowner permission.
On Estancia Tres Hermanos, we sampled ten ideal boulders from two closely-spaced moraines. Estancia Rosa Irene contained a younger moraine set than those found at Estancia Tres Hermanos, and we took twenty-three samples from this location.For the purposes of cosmogenic surface exposure dating, an ideal boulder should be in place, and have its original surface. In addition, the boulders should be shielded only minimally. Corrections can be made for shielding effects, but to introduce the least error, our field crew tried to select boulders where shielding was minimal. After each boulder was approved by the field team, a surface-slab was taken using either a small charge or a set of wedges and shims. The surface characteristics of the boulder and its geomorphological setting were then recorded in field notebooks, photographed, and logged as points on a Trimble GPS.
Conclusions: The samples collected at Bahía Inútil are currently being processed at Lamont-Doherty Earth Observatory for 10Be surface-exposure dating. When geochemical processing at LDEO is complete, the samples will be sent to Lawrence-Livermore National Laboratory, where the 10Be/9Be ratio will be measured. This dating method is highly precise, and should result in a new chronology of glacial recession in Tierra del Fuego, allowing for the testing of larger hypotheses that aim to solve the mystery of the ice ages.
This field excursion was made possible by the generous support of the Churchill Fund- Thank you!
Holocene climate change – McMurdo Sound region, Antarctica –Scott Braddock, Brenda Hall
[2012-present]
Field Team Members – University of Maine (Brenda Hall, Scott Braddock, Audra Norvaisa) University of California – Santa Cruz (Paul Koch, Rachel Brown, Jon Nye)
The aim of this project is to create a Holocene water temperature reconstruction for the Ross Sea region of Antarctica using oxygen isotopes from scallop shells (Adamussium colbecki). Shells found in raised marine terraces and deltas span from the late to mid Holocene, roughly the last 6000 years. Results from this study will provide a proxy for fluctuations in water temperature after the retreat of grounded ice in the Ross Sea. Samples were collected along the Scott Coast in January, 2013, with a field team consisting of three members from the University of Maine and three members from the University of California – Santa Cruz (UCSC). I will use oxygen isotope analysis and radiocarbon dating of the scallop shells to develop this temperature reconstruction and travel to UCSC’s stable isotope laboratory over the summer to begin analyzing data.
An understanding of temperature variations in the Ross Sea region during the Holocene will provide better insight into how the West Antarctic Ice Sheet and local alpine glaciers may react to changing climate in the future.
Funding provided by the National Science Foundation.
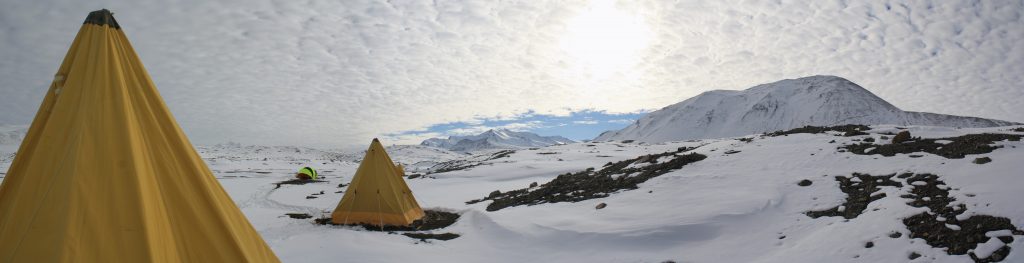
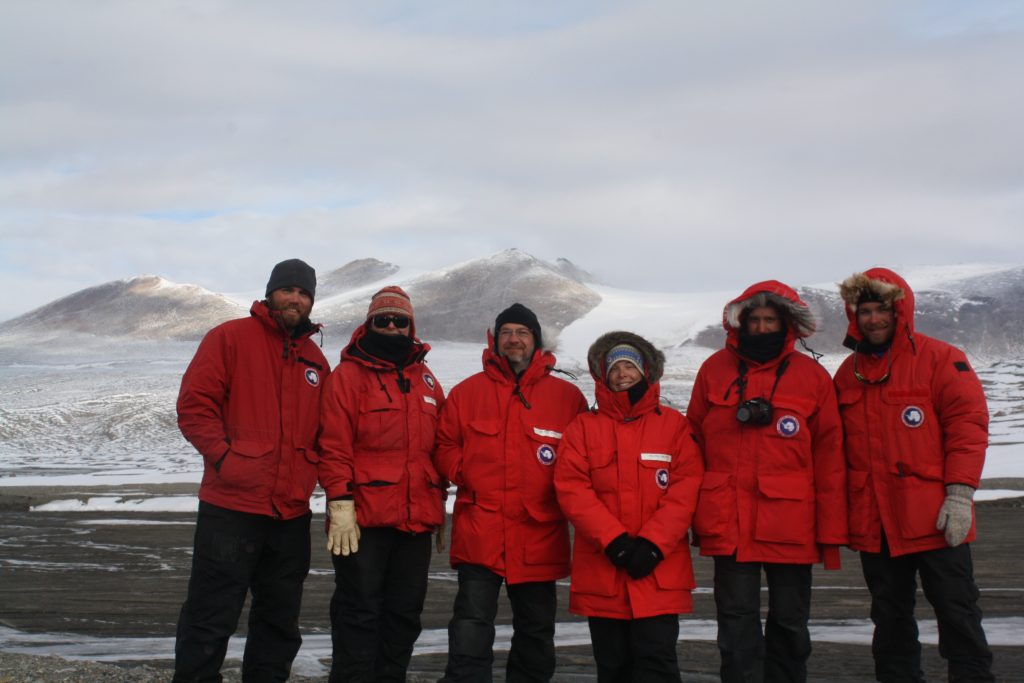
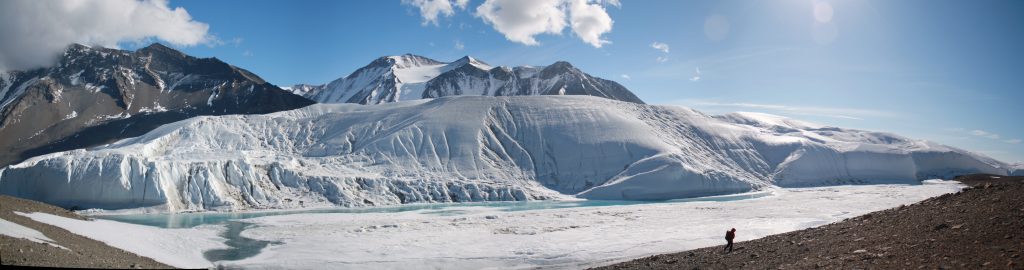
Salmon Valley, Antarctica – Margaret Jackson, Brenda Hall
[2011-present]
During the Last Glacial Maximum, a grounded ice sheet filled the Ross Sea. This ice was thick enough to flow onto the Victoria Land coast and intrude into the valleys of the Royal Society Range. While we know quite a bit about the extent of this ice sheet, the timing of its retreat is more enigmatic. Because of this, Antarctica’s contribution to past sea-level change is an open question.
I’m investigating the history of ice in the Ross Sea, as well as Antarctica’s role in past sea-level change, through study of the glacial history of Salmon Valley in the Royal Society Range. I use glacial geologic mapping and radiocarbon dating of fossil algae to determine the chronology of glaciation and to reconstruct past ice extent.
This work was funded by the National Science Foundation.
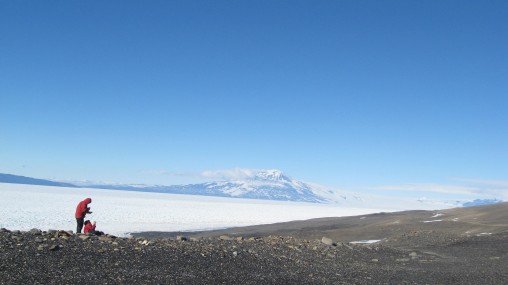
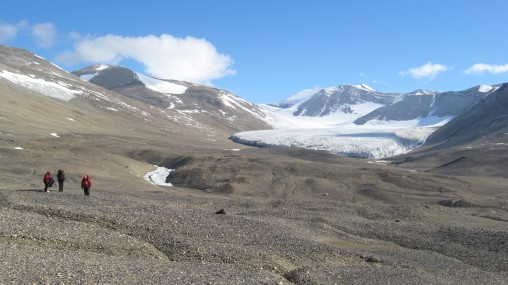